17.2 Review of Anatomy & Physiology – Normal Fetal and Neonatal Circulation
Fetal Circulation
The fetal heart begins beating 21 days after conception, and fetal circulation is established by the fourth week of embryonic life. As the embryo grows within the mother’s uterus, its requirements for nutrients and gas exchange also grow. However, instead of relying on its lungs for oxygenation and its gastrointestinal system for nutrients, the fetus receives oxygenated blood with nutrients from the mother through the umbilical vein from the placenta. After the blood circulates through the fetal body, waste products and carbon dioxide are sent back through two umbilical arteries to the placenta for elimination by the mother.[1]
There are three fetal shunts (i.e., alternate paths for blood flow) in the fetal circulatory system. See Figure 17.1[2] for an illustration of fetal shunts. These shunts are critical for transporting oxygenated blood from the placenta while the fetal lungs are compressed, filled with amniotic fluid, and nonfunctional[3],[4]:
- The ductus venosus is a temporary vessel that connects the umbilical vein to the fetal inferior vena cava. It is a vital part of the fetal circulatory system, allowing oxygenated blood from the placenta to bypass the fetal liver and flow directly to the fetal heart. This enables the oxygenated blood from the placenta to be transported directly to the fetal brain and coronary arteries, the two most metabolically active organs in the developing fetus.
- The foramen ovale is an opening that allows most of this oxygenated blood to flow directly from the fetal right atrium to the left atrium, so the left ventricle can pump it into the aorta and deliver it to the fetal brain and the rest of its body. A valve in this opening prevents backflow of blood during the fetal period.
- The ductus arteriosus is a short vessel that connects the fetal pulmonary trunk to the aorta. Because the fetal lungs are compressed and are not used for air exchange, only a small amount of oxygenated blood is needed to perfuse the lung tissue. The ductus arteriosus diverts most of the already oxygenated blood that is pumped into the pulmonary artery back into the aorta, where it can be delivered to the rest of the body.
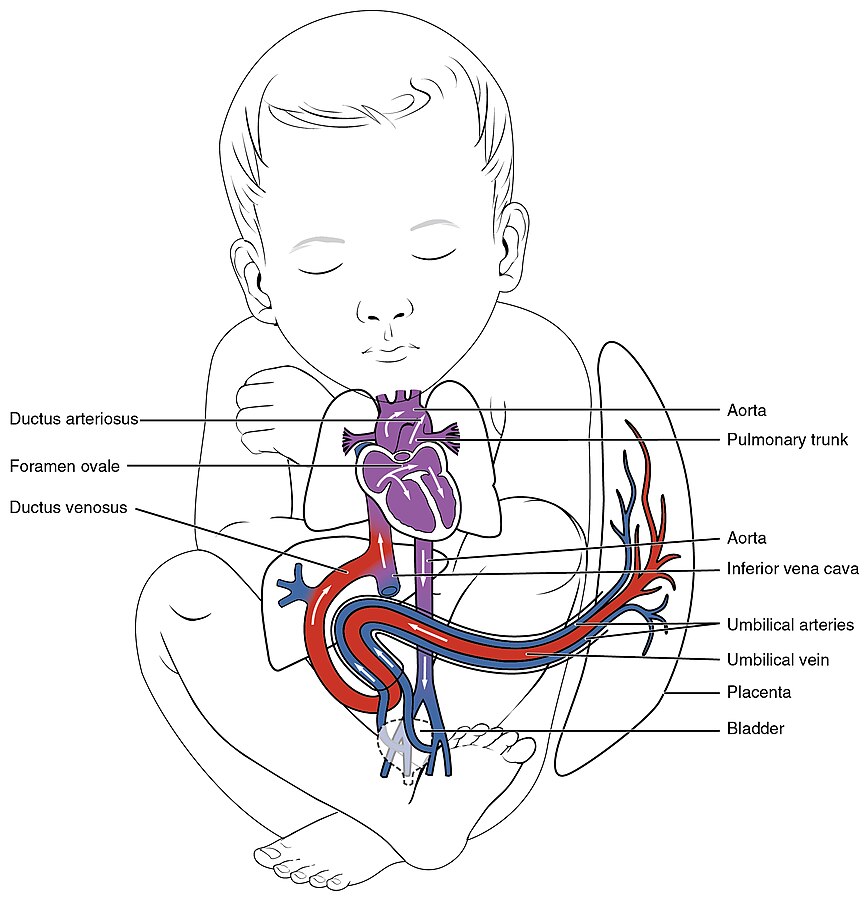
View a supplementary YouTube video[5] explaining fetal circulation: Fetal Circulation by L. McCabe | OPENPediatrics.
Transition to Neonatal Circulation
At birth, the neonate’s first breath initiates the transition away from fetal circulation. When the umbilical cord is clamped, the placenta is removed from the systemic circulation, and systemic vascular resistance begins to rise. As the lungs inflate with air and the alveoli expand, the vessels around them expand in response to the presence of oxygen. Pulmonary pressures begin to decrease, which facilitates increased blood flow through the pulmonary arteries. Gas exchange begins to occur at the alveoli. As a result of the changes in pressures in the heart and lungs, blood no longer flows through the fetal shunts[6],[7]:
- Foramen ovale: When the newborn takes its first breaths and the lungs expand, the blood pressure in the atria increases, causing this shunt to close. The fossa ovalis, a depression in the interatrial septum, remains after birth, marking the location of the former foramen ovale.
- Ductus arteriosus: When the newborn takes its first breaths and the lungs expand, the pressure within the lungs drops dramatically, and the pulmonary vessels expand. The smooth muscles in the wall of the ductus arteriosus constrict, sealing off the passage. Eventually, the muscular and endothelial components of the ductus arteriosus degenerate, leaving only the connective tissue component of the ligamentum arteriosum.
- Ductus venosus: The ductus venosus closes slowly during the first weeks after birth and degenerates to become the ligamentum venosum.
Normal Neonatal Heart Anatomy and Physiology
It’s important to understand the normal anatomy and physiology of the neonatal heart before learning about congenital heart defects. See Figure 17.2[8] for an illustration of the anatomy of a normal heart. This anterior view of the heart shows the four chambers (the right and left atria and the right and left ventricles), the major blood vessels and their branches, and the heart valves. The atrial septum divides the right and left atria, and the ventricular septum divides the right and left ventricles. Note the location of the major vessels, including the aorta, superior vena cava, inferior vena cava, pulmonary arteries, and pulmonary veins. Recall that the right ventricle pumps blood into the pulmonary trunk, which divides into the right and left pulmonary arteries that transport deoxygenated blood to the lungs for oxygenation. After oxygenation occurs in the lungs, the pulmonary veins transport oxygenated blood from the lungs back to the left atrium, where the left ventricle pumps it out to the body through the aorta.[9]
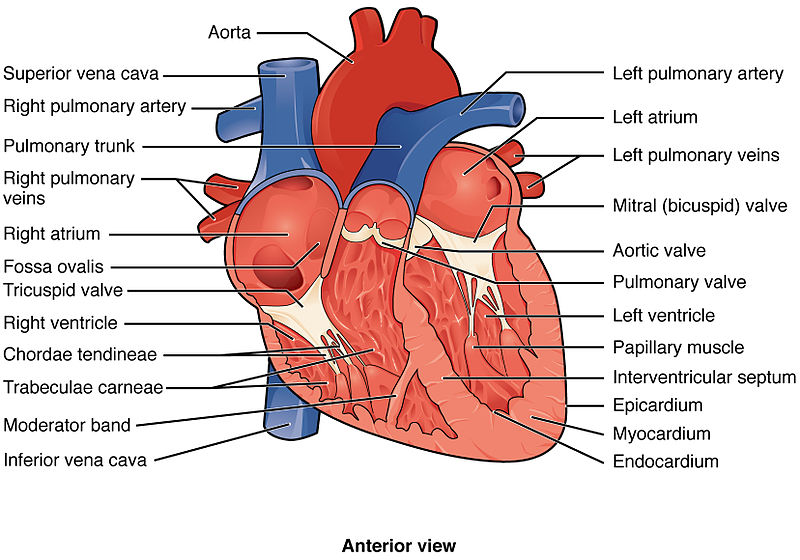
Cardiac output is the volume of blood pumped through the left ventricle over a specified time, usually expressed as liters per minute. Cardiac output is determined by stroke volume, which is the amount of blood ejected with each cardiac cycle (usually expressed as milliliters per heartbeat) multiplied by the heart rate. Stroke volume is affected by contractility, preload, and afterload. Contractility is the force of contraction of the heart muscle. Preload is the degree to which the left ventricle stretches due to fluid volume just before the ventricle contracts. Afterload is the amount of pressure the left ventricle must overcome to eject blood out of the heart and to the rest of the body. Systemic vascular resistance (SVR) is the amount of force exerted by the vasculature of the body, which contributes to afterload.[10]
Neonatal Cardiac Considerations
Newborns up to six months of age have limited capacity to increase their cardiac output in response to hemodynamic changes (i.e., decreased blood pressure) for several reasons[11],[12],[13],[14]:
- A decreased number of mature myocytes (heart muscle cells) results in a decreased ability to stretch (compliance) and increase the force of contractility. This means that a neonate can only minimally increase stroke volume in response to increased cardiac demand. Because of their limited ability to increase stroke volume, neonates rely heavily on their heart rate to maintain cardiac output.
- Newborns have an immature sympathetic nervous system with fewer beta-adrenergic receptors on the heart muscle cells, making it harder for their heart rate and contractility to increase in response to adrenaline (epinephrine). The stress response in newborns, such as response to painful stimuli, can cause bradycardia due to their predominant parasympathetic nervous system tone. Bradycardia can lead to a significant drop in cardiac output and blood pressure because of their reliance on heart rate to maintain cardiac output.
- A newborn’s heart muscle depends primarily on blood glucose to provide energy for contractions. Hypoglycemia reduces contractility, heart rate, and cardiac output. Neonates are at risk for hypoglycemia for several reasons, such as decreased glycogen stores in liver, hypothermia that can result from poor thermoregulation, and the transition from using maternal glucose to metabolizing glucose from food. After the first week of life, the newborn heart becomes more reliant on fatty acids for energy production as the cardiac cells begin to grow in size and mass.
- The neonate’s sarcoplasmic reticulum (SR) is still developing in their cardiac muscle. The SR is responsible for releasing and storing calcium ions in muscle fibers, causing muscles to contract and relax. This affects the contractility of the newborn heart. Neonates cannot effectively store or regulate calcium in their heart muscle cells, making them reliant on serum calcium levels for muscle contraction. Hypocalcemia can cause a variety of complications, such as arrhythmias, poor feeding, muscle weakness, and seizures.
- The newborn’s immature heart muscle is more vulnerable to hypoxia and acidosis, which can further impair their cardiac function.
Circulation Considerations in Newborns
See Table 17.2 for a comparison of normal pediatric heart rate and blood pressure ranges by age. Note that heart rate decreases and blood pressure increases with age as myocardial cells mature.
Table 17.2 Normal Pediatric Heart Rate and Blood Pressure Ranges By Age[15]
Age | HR (beats/minutes) | BP (mmHg) |
Preterm | 120-170 | 40-75/30-35 |
Neonate | 120-160 | 60-75/45-55 |
1-6 months | 100-140 | 70-90/50-65 |
6-12 months | 90-120 | 80-100/55-65 |
Preload Considerations
If the umbilical cord is clamped before the neonate’s lungs have effectively inflated and pulmonary blood flow is established, there is a sudden decrease in preload due to reduced venous return to the left and right ventricles, causing a reduction in cardiac output. Cardiac output is restored after the lungs aerate and pulmonary blood flow increases.
Because neonatal myocardial cells are less compliant, they are not able to effectively stretch to accommodate increases in preload caused by medical interventions such as intravenous fluid boluses. Newborns are at risk for overdistention of the ventricles from rapid fluid increases, placing them at risk for acute heart failure from fluid overload.[16],[17]
Read more information about neonatal heart failure in the “Complications of Congenital Heart Defects” section.
Afterload Considerations
Sudden increases in afterload can significantly affect a newborn’s cardiac output due to decreased compliance in immature heart muscle cells and their reduced capacity to increase stroke volume to overcome increases in afterload.[18],[19]
Heart Rate and Cardiac Output
Neonatal cardiac output is heart rate dependent, meaning the newborn’s heart rate must increase to maintain cardiac output because myocardial cells cannot significantly alter stroke volume. However, as a neonate’s heart rate increases to try to maintain cardiac output, there is less time for the ventricles to fill adequately. Poor ventricular filling can result in decreased perfusion of tissues and organs, even if blood pressure remains normal because of the elevated heart rate. This is important to understand because newborns can be experiencing shock from poor perfusion of their tissues even though their blood pressure is normal. For this reason, decreased blood pressure is a late sign of decompensated shock in neonates.[20]
- Betts, J. G., Desaix, P., Johnson, E., Johnson, J. E., Korol, O., Kruse, D., Poe, B., Wise, J., Womble, M. D., & Young, K. A. (2022). Anatomy and physiology, 2e. OpenStax. https://openstax.org/details/books/anatomy-and-physiology-2e ↵
- “2139_Fetal_Circulation” by OpenStax College is licensed under CC BY 3.0 ↵
- Betts, J. G., Desaix, P., Johnson, E., Johnson, J. E., Korol, O., Kruse, D., Poe, B., Wise, J., Womble, M. D., & Young, K. A. (2022). Anatomy and physiology, 2e. OpenStax. https://openstax.org/details/books/anatomy-and-physiology-2e ↵
- Singh, R. K., & Singh, T. (2022). Heart failure in children: Management. UpToDate. https://www.uptodate.com ↵
- OPENPediatrics. (2021, March 16). Fetal circulation by L. McCabe | OPENPediatrics [Video]. YouTube. All rights reserved. https://www.youtube.com/watch?v=HVBu9HhTkD4 ↵
- Betts, J. G., Desaix, P., Johnson, E., Johnson, J. E., Korol, O., Kruse, D., Poe, B., Wise, J., Womble, M. D., & Young, K. A. (2022). Anatomy and physiology, 2e. OpenStax. https://openstax.org/details/books/anatomy-and-physiology-2e ↵
- Singh, R. K., & Singh, T. (2022). Heart failure in children: Management. UpToDate. https://www.uptodate.com ↵
- “2008_Internal_Anatomy_of_the_HeartN” by OpenStax College is licensed under CC BY 3.0 ↵
- Betts, J. G., Desaix, P., Johnson, E., Johnson, J. E., Korol, O., Kruse, D., Poe, B., Wise, J., Womble, M. D., & Young, K. A. (2022). Anatomy and physiology, 2e. OpenStax. https://openstax.org/details/books/anatomy-and-physiology-2e ↵
- Ord, H., & Griksaitis, M. (2019). Cardiac output diversity: Are children just small adults? Physiology News, (115), 39–41. https://doi.org/10.36866/pn.115.39 ↵
- OpenAnesthesia. (2023). Neonatal cardiovascular physiology. https://www.openanesthesia.org/keywords/neonatal-cardiovascular-physiology/ ↵
- Ord, H., & Griksaitis, M. (2019). Cardiac output diversity: Are children just small adults? Physiology News, (115), 39–41. https://doi.org/10.36866/pn.115.39 ↵
- Bansal, M. (2018). Myocardial disorders in the neonate. NeoReviews, 19(7), e403–e409. https://doi.org/10.1542/neo.19-7-e403 ↵
- Ritterhoff, J., & Tian, R. (2017). Metabolism in cardiomyopathy: Every substrate matters. Cardiovascular Research, 113(4), 411–421. https://doi.org/10.1093/cvr/cvx017 ↵
- OpenAnesthesia. (2023). Neonatal cardiovascular physiology. https://www.openanesthesia.org/keywords/neonatal-cardiovascular-physiology/ ↵
- OpenAnesthesia. (2023). Neonatal cardiovascular physiology. https://www.openanesthesia.org/keywords/neonatal-cardiovascular-physiology/ ↵
- O'Keefe, E., & Singh, P. (2022). Physiology, cardiac preload. StatPearls [Internet]. https://www.ncbi.nlm.nih.gov/books/NBK541109 ↵
- Ord, H., & Griksaitis, M. (2019). Cardiac output diversity: Are children just small adults? Physiology News, (115), 39–41. https://doi.org/10.36866/pn.115.39 ↵
- LaCombe, P., Tariq, M. A. & Lappin. S. L. (2023). Physiology, afterload reduction. StatPearls [Internet]. https://www.ncbi.nlm.nih.gov/books/NBK493174/ ↵
- Ord, H., & Griksaitis, M. (2019). Cardiac output diversity: Are children just small adults? Physiology News, (115), 39–41. https://doi.org/10.36866/pn.115.39 ↵
The umbilical vein is a blood vessel in the umbilical cord that carries oxygenated blood and nutrients from the placenta to the fetus.
A pair of vessels that runs within the umbilical cord and carries fetal blood low in oxygen and high in waste to the placenta for exchange with maternal blood.
An alternate pathway for blood flow in fetal circulation that closes with the infant’s first breaths or within the first weeks after birth as pressure gradients in the circulatory and respiratory system change.
A shunt that carries oxygenated blood from the umbilical vein to the right atrium of the heart in a fetus, bypassing the liver.
A shunt that connects the right atria to the left atria and avoids the pulmonary system altogether.
A shunt within the pulmonary artery which diverts blood into the aorta.
The volume of blood pumped through the left ventricle over a specified time, usually expressed as liters per minute. Cardiac output equals stroke volume x heart rate.
The amount of blood ejected with each cardiac cycle (heart beat).
The force of contraction of the heart muscle.
Filling pressure of the heart.
The amount of pressure the left ventricle must overcome to eject blood out of the heart and to the rest of the body.
The amount of force exerted by the vasculature of the body, which contributes to afterload.
Decreased ability to stretch.