9.3 Anatomy of the Endocrine System
Anatomy of the Endocrine System[1]
Endocrine Glands
The endocrine system consists of cells, tissues, and organs that secrete hormones as a primary or secondary function. Endocrine glands are the primary structural and functional units of the endocrine system. They are considered ductless glands because they secrete their hormones directly into the surrounding fluid rather than through a duct to the surface of the cells or body. The interstitial fluid and the blood vessels then transport the hormones throughout the body to specific target cells.
The endocrine system includes many organs that secrete hormones to regulate homeostasis, such as the pituitary, thyroid, pancreas, parathyroid, adrenal glands, pineal gland, ovaries, and testes. See Figure 9.1[2] for an illustration of the endocrine system and its major endocrine glands. The hypothalamus, thymus, heart, kidneys, stomach, small intestine, liver, and skin are also organs that contain cells with endocrine functions. In addition, adipose tissue produces hormones, and bone tissue also has endocrine functions.
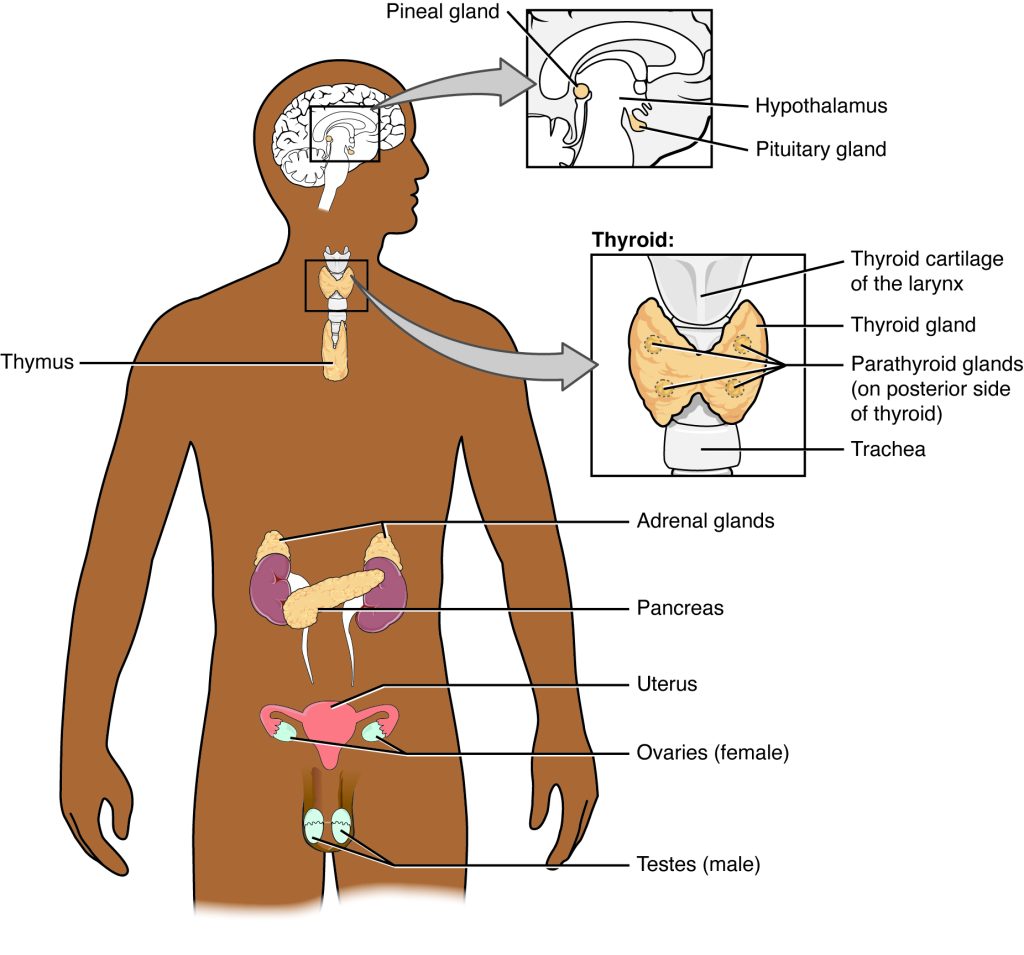
As mentioned earlier, endocrine glands release their products (hormones) directly into the bloodstream, whereas exocrine glands release their secretions through ducts. Examples of exocrine glands include the sebaceous (oil) glands and sudoriferous (sweat) glands of the skin. Some organs serve as both endocrine and exocrine organs. For example, the pancreas secretes pancreatic juice into the small intestine as part of the digestive process, but it also has an endocrine function of secreting insulin and glucagon into the blood to regulate blood glucose levels.
Hormone Target Cells
Although a hormone travels throughout the body in the bloodstream, it only affects its specific target cells that are cells with receptors for that particular hormone. A receptor is a protein located either inside the cell or within the cell’s membrane that binds a hormone, initiating the target cell response. After the hormone binds to the receptor, a chain of events leads to the target cell’s response. Different hormones contribute to many different physiological responses in the body, including human reproduction, growth and development of body tissues, metabolism, fluid and electrolyte balance, sleep, and many other body functions. We will review several hormones and their actions later in this chapter. The major hormones of the human body and their effects are identified in Table 9.3a.
Table 9.3a. Endocrine Glands and Their Major Hormones[3]
Endocrine Gland | Hormones | Effects |
---|---|---|
Pituitary (anterior) | Human growth hormone (hGH) | Promotes growth of body tissues |
Pituitary (anterior) | Prolactin (PRL) | Promotes milk production |
Pituitary (anterior) | Thyroid-stimulating hormone (TSH) | Stimulates thyroid hormone secretion by thyroid gland |
Pituitary (anterior) | Adrenocorticotropic hormone (ACTH) | Stimulates hormone secretion by adrenal cortex |
Pituitary (anterior) | Follicle-stimulating hormone (FSH) | Stimulates gamete production |
Pituitary (anterior) | Luteinizing hormone (LH) | Stimulates sex hormone secretion by gonads and ovulation |
Pituitary (posterior) | Antidiuretic hormone (ADH) | Stimulates water reabsorption by kidneys |
Pituitary (posterior) | Oxytocin | Stimulates uterine contractions during childbirth and milk letdown |
Thyroid | Thyroxine (T4) and triiodothyronine (T3) | Stimulates basal metabolic rate |
Thyroid | Calcitonin | Decreases blood Ca2+ levels |
Parathyroid | Parathyroid hormone (PTH) | Increases blood Ca2+ levels |
Adrenal (cortex) | Aldosterone | Increases blood Na+ levels, water reabsorption, and K+ excretion by kidneys |
Adrenal (cortex) | Cortisol, corticosterone, and cortisone | Increases blood glucose levels during the stress response, reduces inflammation, and in high amounts suppresses immunity |
Adrenal (medulla) | Epinephrine, norepinephrine | Stimulates fight-or-flight response |
Pineal | Melatonin | Regulates sleep cycles |
Pancreas | Insulin | Decreases blood glucose levels |
Pancreas | Glucagon | Increases blood glucose levels |
Testes | Testosterone | Stimulates development of sex characteristics, including a deeper voice, increased muscle mass, development of body hair, and sperm production |
Ovaries | Estrogens and progesterone | Stimulates development of sex characteristics, including the development of adipose and breast tissue, regulates menstrual cycles, and prepares the body for childbirth |
Types of Hormones
The hormones of the human body can be categorized into two major groups based on their chemical structure as either water-soluble or fat-soluble. These chemical groups affect a hormone’s distribution, the type of receptors it binds to, and other aspects of its function.
Hormones derived from amino acids include amines, peptides, and proteins and are also known as water-soluble hormones. They cannot cross through the phospholipid cell membrane, as they are repelled by the fatty interior of the membrane. They bind to receptors on the outside of the cell membrane that will facilitate a cascade of events inside the cell to activate certain proteins.
Hormones derived from lipids are fat-soluble and include steroids. Because they are fat soluble, they can cross through the cell membrane and bind to receptors inside the cell. Lipid-soluble hormones are not soluble in water (they are hydrophobic). Because blood is water-based, lipid-soluble hormones must travel to their target cell bound to a transport protein.
Pathways of Hormone Action
The message a hormone sends is received by a hormone receptor, a protein located either inside the cell or within or on the cell membrane. The receptor will receive the message and initiate the target cell’s response. Hormone receptors are selective and only bind to hormones with a specific shape or structure that matches the receptor. The same type of receptor may be located on cells in different body tissues and trigger somewhat different responses. Therefore, the response triggered by a hormone depends not only on the hormone, but also on the target cell.
After the target cell receives the hormone signal, it can respond in a variety of ways. The response may include the stimulation of protein synthesis, activation or deactivation of enzymes, alteration in the permeability of the cell membrane, altered rates of mitosis and cell growth, or the stimulation of the secretion of products. Moreover, a single hormone may be capable of inducing different responses in a given cell.
Factors Affecting Target Cell Response
As mentioned earlier, target cells must have receptors specific to a given hormone if that hormone is to trigger a response. However, several other factors influence the target cell response. For example, the presence of a significantly high level of a hormone circulating in the bloodstream can cause its target cells to decrease their number of receptors for that hormone. This process is called downregulation, and it allows cells to become less reactive to the excessive hormone levels.
When the level of a hormone is chronically reduced, target cells engage in upregulation to increase their number of receptors. This process allows cells to be more sensitive to the hormone that is present. Cells can also alter the sensitivity of the receptors themselves to various hormones.
Two or more hormones can interact to affect the response of cells in a variety of ways. The three most common types of interactions are as follows:
- The permissive effect, in which the presence of one hormone enables another hormone to act. For example, thyroid hormones have complex permissive relationships with certain reproductive hormones. A dietary deficiency of iodine, a component of thyroid hormones, can, therefore, affect reproductive system development and functioning.
- The synergistic effect, in which two hormones with similar effects produce an amplified or bigger response together. In some cases, two hormones are required for an adequate response. For example, two different reproductive hormones—FSH from the pituitary gland and estrogens from the ovaries—are required for the maturation of female ova (egg cells).
- The antagonistic effect, in which two hormones have opposing effects. A familiar example is the effect of two pancreatic hormones, insulin and glucagon. Insulin decreases blood glucose, whereas glucagon increases blood glucose.
Regulation of Hormone Secretion
To prevent abnormal hormone levels and a potential disease state, hormone levels must be tightly controlled. The body maintains this control by balancing hormone production and breakdown with positive and negative feedback loops.
Positive Feedback Loops
Recall that positive feedback loops amplify or exaggerate the original stimulus. Positive feedback loops in hormonal regulation occur when the release of a hormone triggers the release of even more of that same hormone. An example of a positive feedback loop is the release of oxytocin during childbirth. The initial release of oxytocin begins to signal the uterine muscles to contract, which pushes the fetus toward the cervix, causing it to stretch. The stretching signals the pituitary gland to release more oxytocin, causing labor contractions to intensify. This continues until the birth of the child when the stretching stops and oxytocin is decreased.
Negative Feedback Loops
The more common method of hormone regulation is the negative feedback loop. Recall that in negative feedback loops, the original stimulus is reduced or stopped. Negative feedback loops in hormonal regulation slow down or stop (inhibit) further secretion of a hormone in response to adequate levels of a hormone or other substance, such as glucose or calcium. This allows blood levels of the hormone to be regulated within a narrow range. An example of a negative feedback loop is the release of glucocorticoid hormones from the adrenal glands, as directed by the hypothalamus and pituitary gland. As glucocorticoid concentrations in the blood rise, the hypothalamus and pituitary gland reduce their signaling to the adrenal glands to prevent additional glucocorticoid secretion. See Figure 9.2[4] for an illustration of this negative feedback loop.
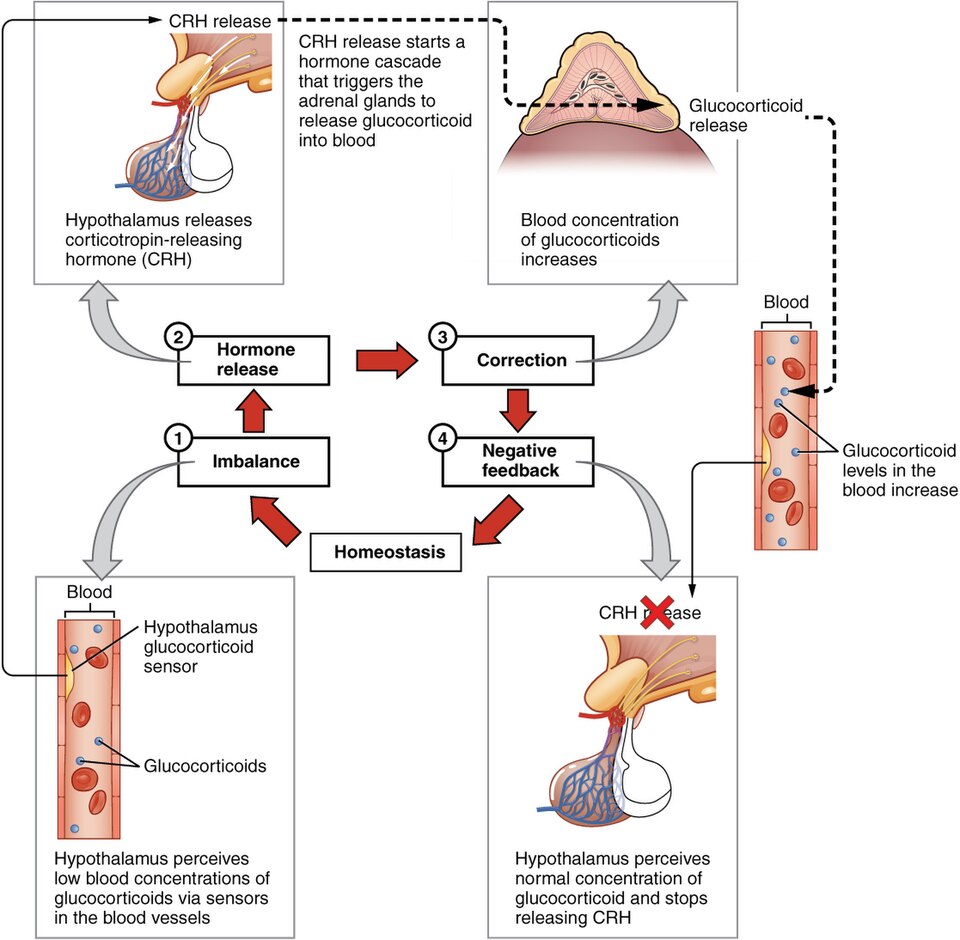
Hypothalamus-Pituitary Complex
The hypothalamus–pituitary complex can be thought of as the “command center” of the endocrine system. This complex secretes several hormones that directly produce responses in target tissues, as well as hormones that regulate the secretion (production and release) of hormones from other glands. In addition, the hypothalamus–pituitary complex coordinates the messages of the endocrine and nervous systems. In many cases, a stimulus received by the nervous system must pass through the hypothalamus–pituitary complex to be translated into hormones that can initiate a response. See Figure 9.3[5] for an illustration of the hypothalamus-pituitary complex.
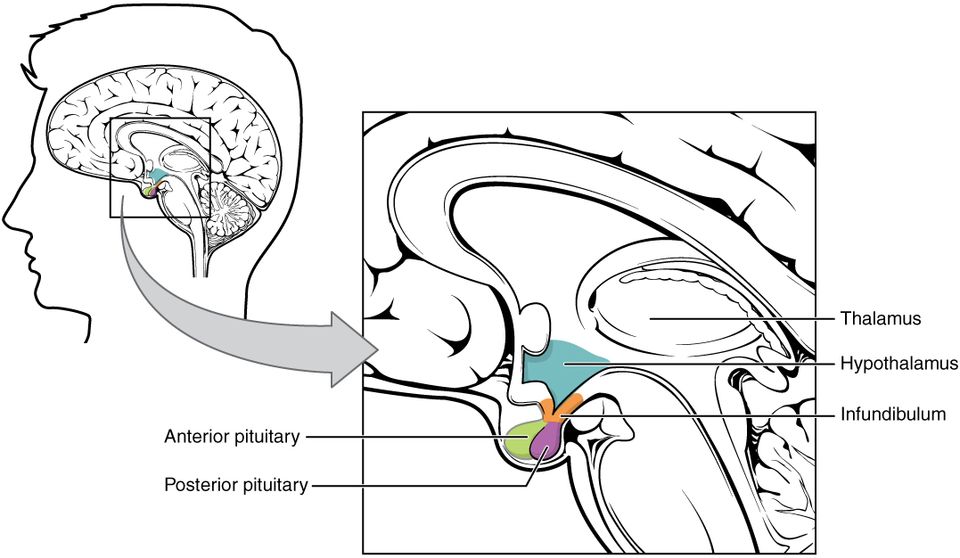
Hypothalamus
The hypothalamus is a structure of the diencephalon of the brain located anterior and inferior to the thalamus. It has both neural and endocrine functions, producing and secreting many hormones.
Pituitary Gland
The hypothalamus is anatomically and functionally related to the pituitary gland (also known as the hypophysis), a pea-sized organ suspended from a stem called the infundibulum (or pituitary stalk). The pituitary gland is cradled within the sella turcica of the sphenoid bone of the skull. It consists of two lobes: the posterior pituitary (also known as the neurohypophysis) and the anterior pituitary (also known as the adenohypophysis) that both release hormones. The pituitary gland also contains the intermediate pituitary, a small group of cells between the lobes that secretes a hormone as well.
Anterior Pituitary Gland
The anterior pituitary gland secretes several hormones. However, the secretion of hormones from the anterior pituitary is regulated by two classes of hormones that are secreted by the hypothalamus called releasing hormones and inhibiting hormones:
- Releasing hormones: The hypothalamus secretes releasing hormones to stimulate the secretion of hormones from the anterior pituitary gland.
- Inhibiting hormones: The hypothalamus secretes inhibiting hormones to slow or stop the secretion of hormones from the anterior pituitary gland.
See Figure 9.4[6] for an illustration of the anterior pituitary gland.
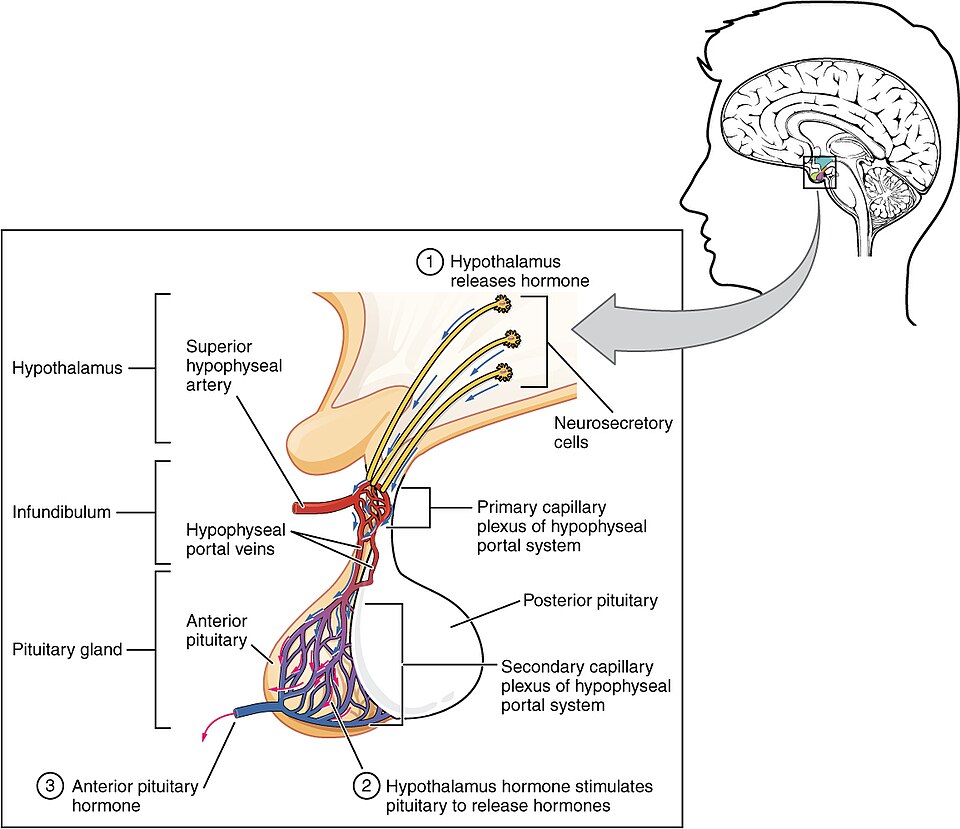
Anterior Pituitary Gland Hormones
The anterior pituitary produces several hormones, including the human growth hormone (hGH), thyroid-stimulating hormone (TSH), adrenocorticotropic hormone (ACTH), follicle-stimulating hormone (FSH), luteinizing hormone (LH), and prolactin (PRL). Of the hormones of the anterior pituitary, TSH, ACTH, FSH, and LH are collectively referred to as tropic hormones (trope- = “turning”) because they turn on or off the function of other endocrine glands.
Human Growth Hormone
The endocrine system regulates the growth of the human body, protein synthesis, and cellular replication. A major hormone involved in this process is the human growth hormone (hGH), also called somatotropin—a protein hormone secreted by the anterior pituitary gland. It promotes protein synthesis and tissue building through direct and indirect mechanisms. See Figure 9.5[7] for an illustration of the release and inhibition of hGH and its effects. hGH levels are controlled by the release of GHRH (growth hormone releasing hormone) and GHIH (growth hormone inhibiting hormone), also known as somatostatin, from the hypothalamus.
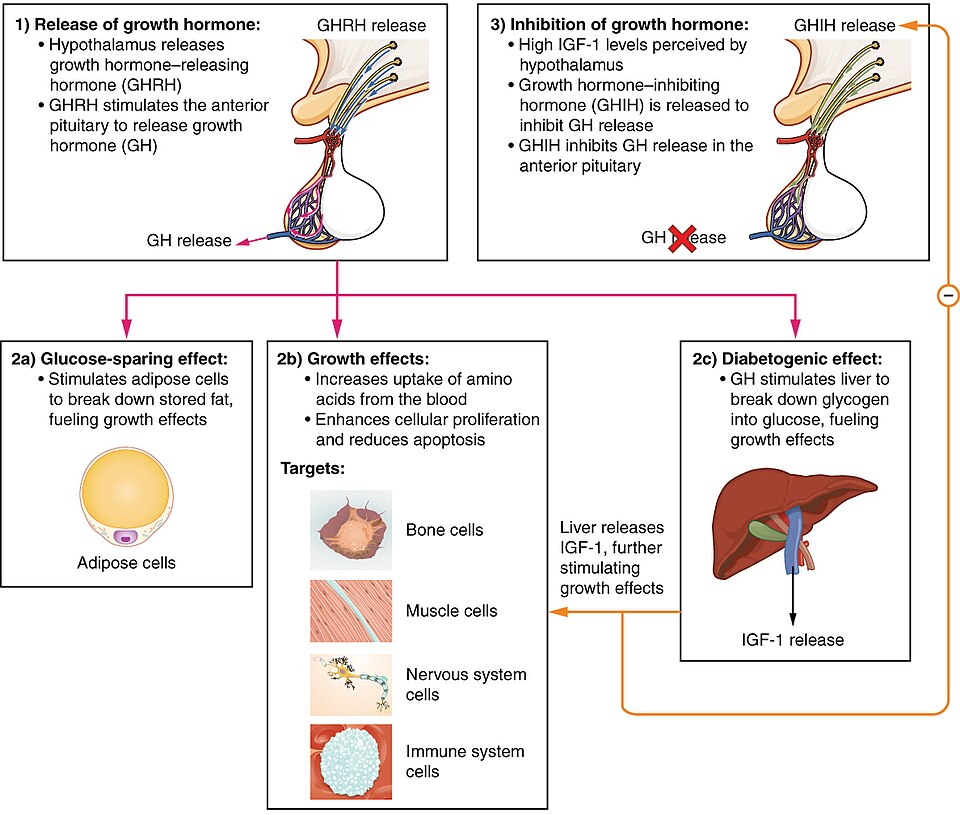
Human growth hormone directly increases the rate of protein synthesis in skeletal muscle and bones. hGH indirectly controls growth and protein synthesis by stimulating the liver and other tissues to produce a group of proteins called insulin-like growth factors (IGFs). These proteins enhance cellular proliferation and inhibit apoptosis, or programmed cell death. IGFs stimulate cells to increase their uptake of amino acids from the blood for protein synthesis. Skeletal muscle and cartilage cells are particularly sensitive to stimulation from IGFs.
Excesses or deficiencies of any hormone can result in several disorders. For example, gigantism is a disorder in children that is caused by the secretion of abnormally large amounts of hGH, resulting in excessive growth. A similar condition in adults is acromegaly, a disorder that results in the thickening of bones in the face, hands, and feet in response to excessive levels of hGH in individuals who have stopped growing. Abnormally low levels of hGH in children can cause growth impairment—a disorder called pituitary dwarfism (also known as growth hormone deficiency).
See Figure 9.6[8] for an image of an individual with gigantism compared to the size of his family members and Figure 9.7[9] for an image of two individuals with dwarfism.
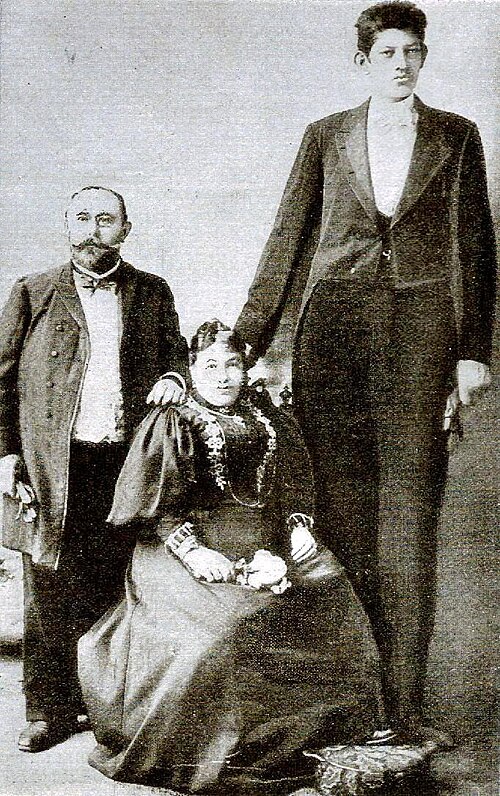
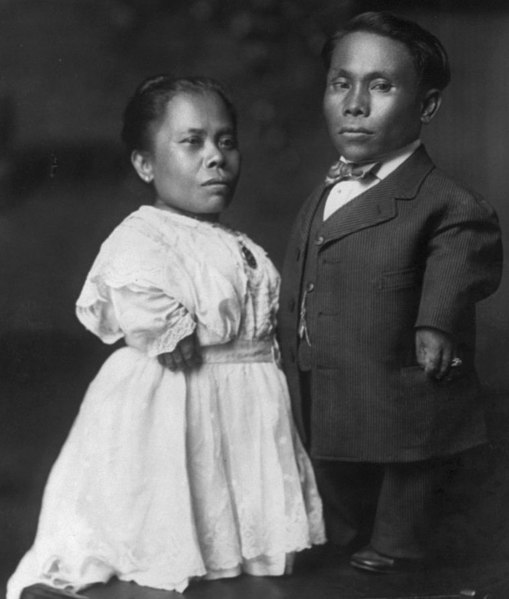
Thyroid-Stimulating Hormone
The activity of the thyroid gland is regulated by thyroid-stimulating hormone (TSH), also called thyrotropin. TSH is released from the anterior pituitary in response to thyrotropin-releasing hormone (TRH) from the hypothalamus and then triggers the secretion of thyroid hormones by the thyroid gland. In a classic negative feedback loop, elevated levels of thyroid hormones in the bloodstream then trigger a drop in production of TRH and, subsequently, TSH.
Adrenocorticotropic Hormone
The adrenocorticotropic hormone (ACTH), also called corticotropin, stimulates the adrenal cortex (the outer layer of the adrenal glands) to secrete corticosteroid hormones such as cortisol. The release of ACTH is regulated by the corticotropin-releasing hormone (CRH) from the hypothalamus.
Follicle-Stimulating Hormone and Luteinizing Hormone
The endocrine glands secrete a variety of hormones that control the development and regulation of the reproductive system. Much of the development of the reproductive system occurs during puberty, which is initiated by gonadotropin-releasing hormone (GnRH), a hormone secreted by the hypothalamus. GnRH stimulates the anterior pituitary to secrete gonadotropins—hormones that regulate the function of the gonads, which are the ovaries in females and the testes in males. Throughout life, gonadotropins regulate reproductive function and, in the case of females, the onset and end of reproductive ability.
The gonadotropins include two hormones: follicle stimulating hormone (FSH) and luteinizing hormone (LH). FSH stimulates the production and maturation of sex cells, or gametes, which are ova and sperm. FSH also promotes follicular growth; follicles are sacs that ova develop in, and these follicles then release estrogens from the ovaries. LH triggers ovulation, as well as the production of estrogens and progesterone by the ovaries. In males, LH stimulates production of testosterone by the testes.
Prolactin
As its name implies, prolactin (PRL) promotes lactation (milk production). During pregnancy, it contributes to development of the mammary glands, and after birth, it stimulates the mammary glands to produce breast milk. However, the effects of prolactin depend heavily on the permissive effects of estrogens, progesterone, and other hormones.
In non-pregnant females, prolactin secretion is inhibited by prolactin-inhibiting hormone (PIH), which is actually the neurotransmitter dopamine released from neurons in the hypothalamus. Only during pregnancy do prolactin levels rise in response to prolactin-releasing hormone (PRH) from the hypothalamus.
Intermediate Pituitary Hormone: Melanocyte-Stimulating Hormone
The cells in between the pituitary lobes secrete a hormone known as melanocyte-stimulating hormone (MSH). Local production of MSH in the skin is responsible for melanin production in response to UV light exposure. The role of MSH made by the pituitary is more complicated. For instance, people with lighter skin generally have the same amount of MSH as people with darker skin. Nevertheless, this hormone is capable of darkening the skin by inducing melanin production in the skin’s melanocytes. People also show increased MSH production during pregnancy; in combination with estrogens, it can lead to darker skin pigmentation, especially the skin of the areolas and labia minora.
Posterior Pituitary Gland Hormones
The posterior pituitary gland does not produce hormones but rather stores and releases two hormones produced by the hypothalamus. These two hormones are oxytocin and ADH (antidiuretic hormone).
Oxytocin
When fetal development is complete, the hormone oxytocin (tocia- = “childbirth”) stimulates uterine contractions and dilation of the cervix. Additionally, oxytocin is necessary for the milk ejection reflex (commonly referred to as “let-down”) in breastfeeding and is thought to contribute to parent–newborn bonding, known as attachment. Oxytocin is also thought to be involved in feelings of love and closeness, as well as in the sexual response for both men and women.
Antidiuretic Hormone (ADH)
The solute concentration of the blood, or blood osmolality, may change in response to the consumption of certain foods and fluids, as well as in response to disease, injury, medications, or other factors. Blood osmolality is constantly monitored by osmoreceptors—specialized cells within the hypothalamus that are particularly sensitive to the concentration of sodium ions and other solutes. The higher the osmolality, the more dehydrated a person can become.
In response to high blood osmolality, which can occur during dehydration or following a very salty meal, the osmoreceptors signal the posterior pituitary to release antidiuretic hormone (ADH). The target cells of ADH are located in the kidneys. Its effect is to allow increased water reabsorption. The more water reabsorbed, the greater the amount of water that is returned to the blood and the less that is excreted in the urine. ADH is also known as vasopressin because, in very high concentrations, it causes constriction of blood vessels, which increases blood pressure.
Interestingly, drugs can affect the secretion of ADH. For example, alcohol consumption inhibits the release of ADH, resulting in increased urine production that can eventually lead to dehydration and a hangover. See Figure 9.8[10] for an illustration of the pituitary hormones and their target organs.
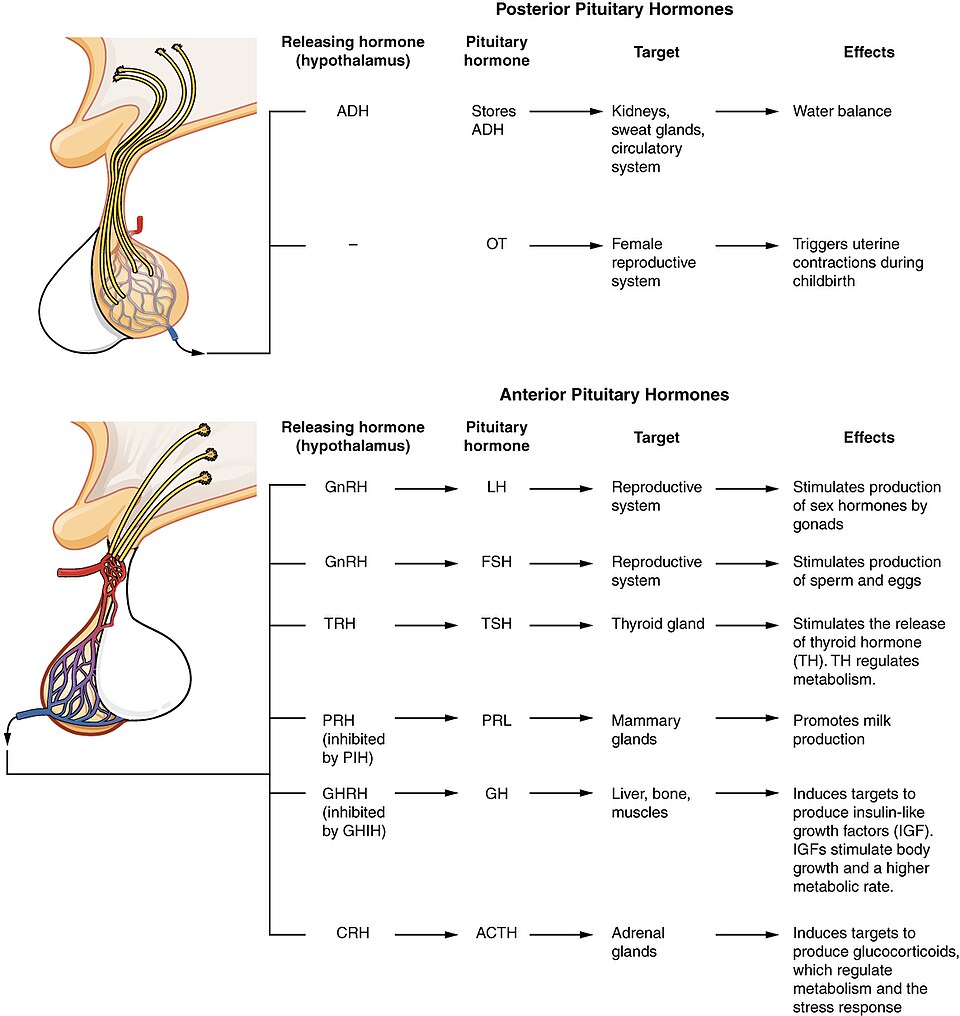
Other Endocrine Glands
Thyroid Gland
A butterfly-shaped organ, the thyroid gland is located anterior to the trachea, just inferior to the larynx in the neck. See Figure 9.9[11] for an illustration of the thyroid gland and an image of thyroid follicle cells. The middle region, called the isthmus, connects the two wing-shaped left and right lobes. Each of the thyroid lobes are embedded with parathyroid glands, primarily on their posterior surfaces.
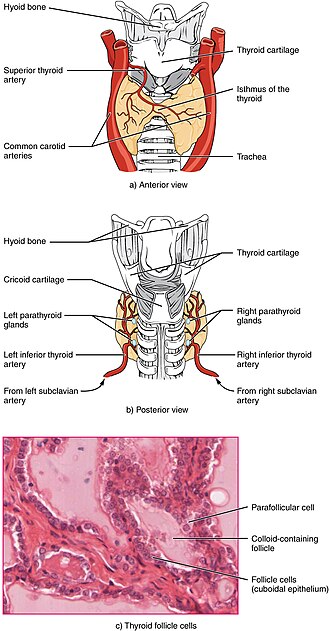
T3 and T4
TSH (thyroid stimulating hormone) from the anterior pituitary gland stimulates the thyroid gland to secrete triiodothyronine (T3), a thyroid hormone with three iodine atoms in its chemical structure and tetraiodothyronine, also known as thyroxine (T4), a thyroid hormone with four iodine atoms in its structure.
As shown in Figure 9.10[12], low blood levels of T3 and T4 stimulate the release of thyrotropin-releasing hormone (TRH) from the hypothalamus, which triggers secretion of TSH from the anterior pituitary. In turn, TSH stimulates the thyroid gland to secrete T3 and T4. Interestingly, the T4 produced by the thyroid gland is converted to T3 by some of the body’s cells.[13] The levels of TRH, TSH, T3, and T4 are regulated by a negative feedback system in which increasing levels of T3 and T4 decrease the secretion of TSH.
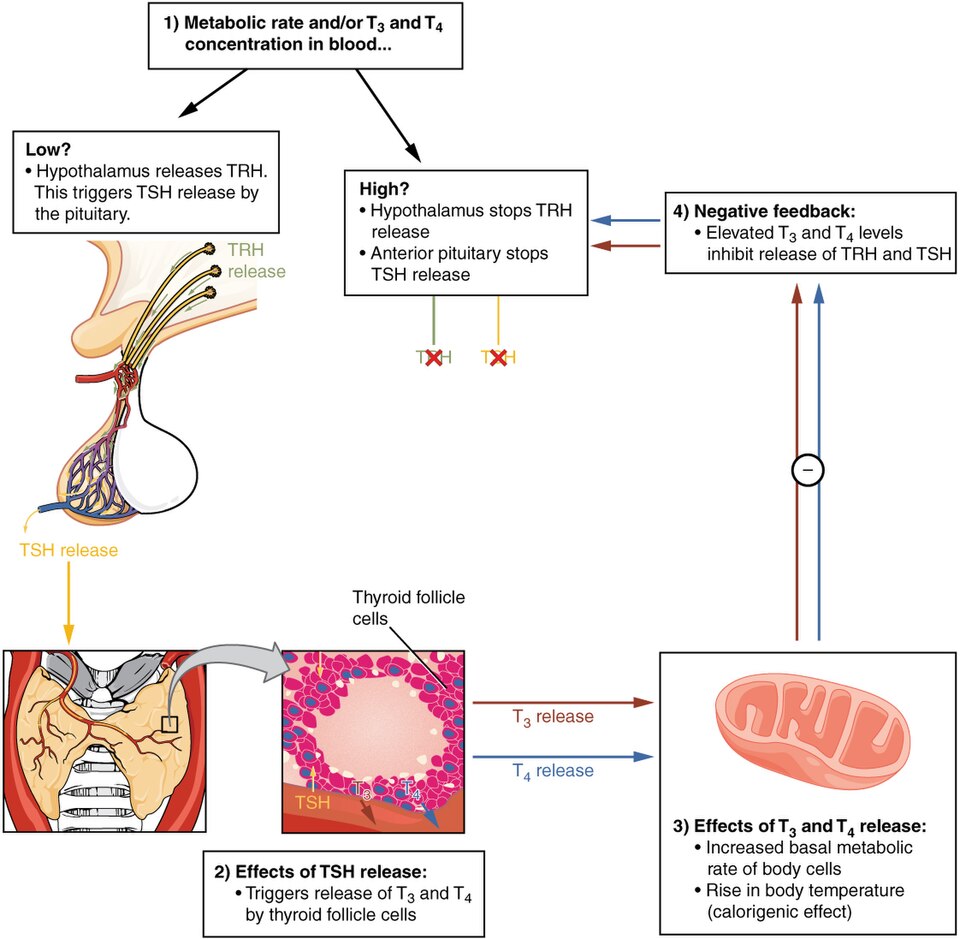
Functions of Thyroid Hormones
The thyroid hormones, T3 and T4, are often referred to as metabolic hormones because their levels influence the body’s basal metabolic rate, the amount of energy used by the body at rest. Adequate levels of thyroid hormones are also required for protein synthesis and for fetal and childhood tissue development and growth. They are especially critical for normal development of the nervous system both in utero and in early childhood, and they continue to support neurological function in adults.
These thyroid hormones also have a complex interrelationship with reproductive hormones, and deficiencies can influence libido, fertility, and other aspects of reproductive function. Finally, thyroid hormones increase the body’s sensitivity to epinephrine and norepinephrine from the adrenal medulla by upregulation of receptors in the blood vessels. When levels of T3 and T4 hormones are excessive, this effect accelerates the heart rate, strengthens the heartbeat, and increases blood pressure. Because thyroid hormones regulate metabolism, heat production, protein synthesis, and many other body functions, thyroid disorders can have severe and widespread consequences.
Calcitonin
The thyroid gland also secretes a hormone called calcitonin that is produced by the parafollicular cells (also called C cells) of the thyroid gland. Calcitonin is released in response to a rise in blood calcium levels. It is thought to decrease blood calcium levels by inhibiting the activity of osteoclasts, bone cells that release calcium into the circulation by breaking down bone and increasing osteoblastic (bone building) activity. It also decreases calcium absorption in the intestines and increases calcium loss in the urine.
The hormones released by the thyroid gland and their effects are summarized in Table 9.3b.
Table 9.3b. Thyroid Hormones[14]
Hormones | Effect |
---|---|
Thyroxine (T4), triiodothyronine (T3) | Stimulates basal metabolic rate |
Calcitonin | Decreases blood Ca2+ levels |
Parathyroid Glands
The parathyroid glands are tiny, round structures usually found embedded in the posterior surface of the thyroid gland. See Figure 9.11[15] for an illustration of the parathyroid glands and an image of parathyroid tissue. A thick connective tissue capsule separates the glands from the thyroid tissue. Most people have four parathyroid glands, but occasionally there are more in tissues of the neck or chest. The primary functional cells of the parathyroid glands are the chief cells, which produce and secrete parathyroid hormone (PTH), the major hormone responsible for increasing blood calcium levels.
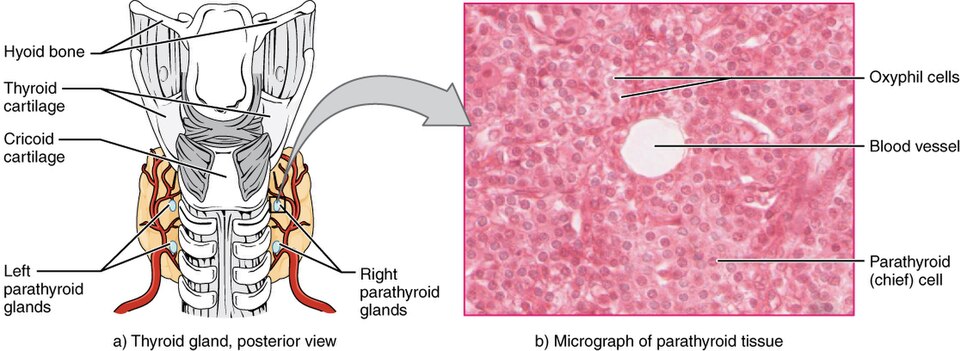
The parathyroid glands produce and secrete PTH in response to low blood calcium levels. PTH secretion causes the release of calcium from the bones by stimulating osteoclasts, which secrete enzymes that break down bone and release calcium into the interstitial fluid. PTH also inhibits osteoblasts, the cells involved in bone building, thereby saving blood calcium. PTH also causes increased reabsorption of calcium (and magnesium) in the kidney from the urine filtrate. In addition, PTH initiates the production of the steroid hormone calcitriol (also known as 1,25-dihydroxyvitamin D), which is the active form of vitamin D3, in the kidneys. Calcitriol then stimulates increased absorption of dietary calcium by the intestines. A negative feedback loop regulates the levels of PTH, with rising blood calcium levels inhibiting further release of PTH. See Figure 9.12[16] for an illustration of this feedback loop.
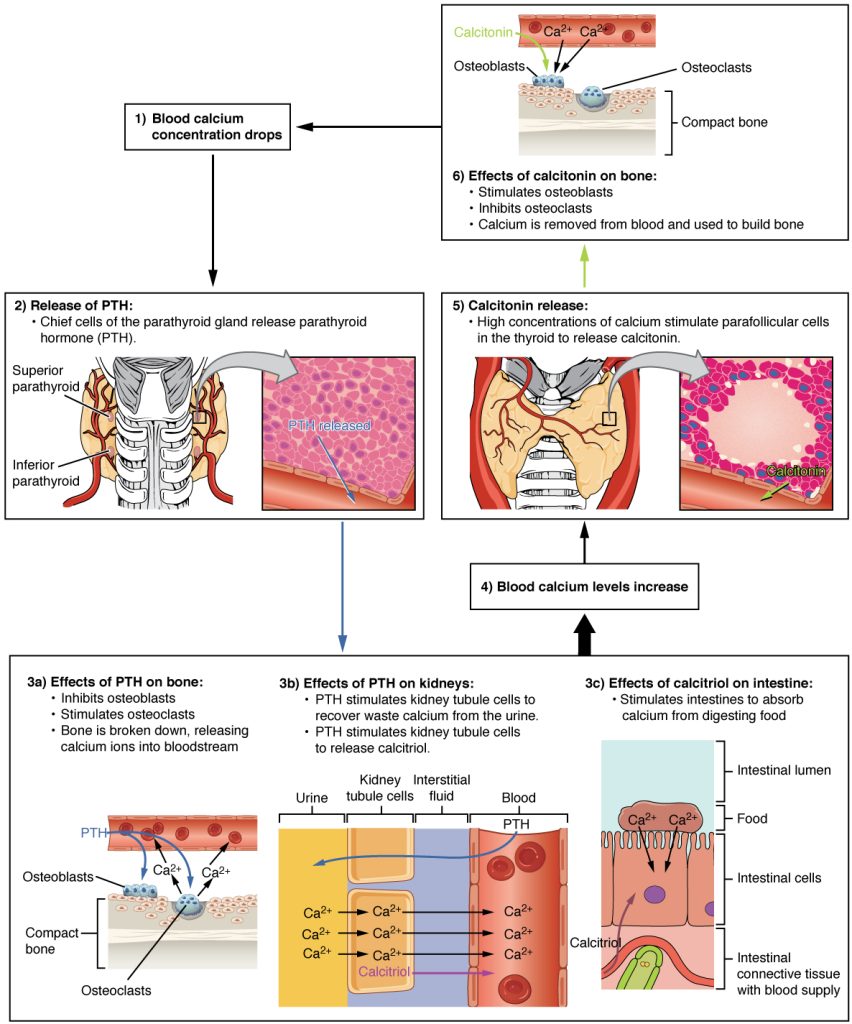
Adrenal Glands
The adrenal glands are wedges of tissue attached to the top of both kidneys by a fibrous capsule. The adrenal gland consists of an outer cortex of glandular tissue and an inner medulla of nervous tissue. The adrenal glands have a rich blood supply and experience one of the highest rates of blood flow in the body. Blood flows to each adrenal gland at the adrenal cortex and then drains into the adrenal medulla. See Figure 9.13[17] for an illustration of the adrenal gland.
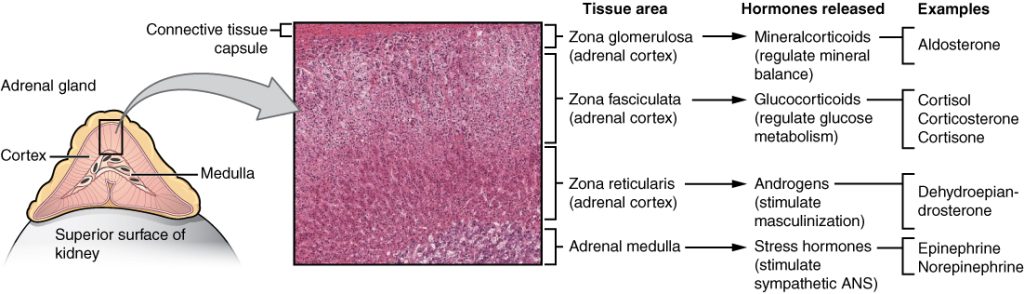
The adrenal cortex is a component of the hypothalamic-pituitary-adrenal (HPA) axis. The HPA axis involves the secretion of corticotropin releasing hormone (CRH) from the hypothalamus, which stimulates the secretion of adrenocorticotropic hormone (ACTH) from the pituitary. ACTH then stimulates the adrenal cortex to secrete steroid hormones, such as cortisol, which are important for the regulation of the long-term stress response, blood pressure and blood volume, nutrient uptake and storage, fluid and electrolyte balance, and inflammation.
The adrenal medulla is neuroendocrine tissue that is an extension of the autonomic nervous system. Impulses from the hypothalamus stimulate the adrenal medulla to secrete the hormones epinephrine and norepinephrine during the fight-or-flight response.
One of the major functions of the adrenal gland is to respond to stress. Stress can be physical, psychological, or both. Physical stressors include illness, injury, malnutrition, or environmental exposures such as being outside in hot, cold, or wet conditions. Psychological stressors include events such as the perception of a physical threat, a fight with a loved one, or a bad day at work or school.
Adrenal hormones also have several non-stress-related functions, including the increase of blood sodium and glucose levels that will be described in detail later in this chapter.
Adrenal Cortex Hormones
The adrenal cortex produces a group of hormones collectively referred to as mineralocorticoids because of their effect on body minerals, especially sodium and potassium. These hormones are essential for fluid and electrolyte balance. Aldosterone is the major mineralocorticoid. It is important in the regulation of sodium and potassium ions in the blood, urine, sweat, and saliva. For example, it is released in response to elevated blood potassium (K+), low blood sodium (Na+), low blood pressure, or low blood volume. In response, aldosterone increases the excretion of K+ and the retention of Na+, which, in turn, increases blood volume and blood pressure.
The adrenal cortex also produces hormones called glucocorticoids because of their role in glucose metabolism. The most important of these is cortisol, some of which the liver converts to cortisone. Their overall effect is to inhibit tissue building while stimulating the breakdown of stored nutrients to maintain adequate fuel supplies. In conditions of long-term stress, for example, cortisol promotes the breakdown of glycogen to glucose, the breakdown of stored triglycerides into fatty acids and glycerol, and the breakdown of muscle proteins into amino acids. These raw materials can then be used to make additional glucose and ketones for use as body fuels.
You may be familiar with prescription and over-the-counter medications containing glucocorticoids, such as cortisone injections into inflamed joints, prednisone tablets and steroid-based inhalers used to manage severe asthma, and hydrocortisone creams applied to relieve itchy rashes. These drugs reflect another role of cortisol—the downregulation of the immune system, which reduces the inflammatory response and in high amounts can suppress immunity.
The adrenal cortex also produces small amounts of steroid sex hormones called androgens. During puberty and most of adulthood, androgens are produced in the gonads (ovaries and testes). The androgens produced in the adrenal cortex supplement the gonadal androgens. They are produced in response to ACTH from the anterior pituitary and are converted in the tissues to testosterone or estrogens. In adult females, they may contribute to the sex drive, but their function in adult males is not well-understood. In postmenopausal females, as the functions of the ovaries decline, the main source of estrogens becomes the androgens produced by the adrenal cortex.
Adrenal Medulla Hormones
The adrenal medulla releases hormones in response to acute, short-term stress mediated by the sympathetic nervous system.
The adrenal medulla produces the hormones epinephrine (also called adrenaline) and norepinephrine (or noradrenaline). Epinephrine is produced in greater quantities—approximately a 4 to 1 ratio with norepinephrine—and is the more powerful hormone.
Both epinephrine and norepinephrine signal the liver and skeletal muscle cells to convert glycogen into glucose, resulting in increased blood glucose levels. These hormones increase the heart rate and blood pressure to prepare the body to fight the perceived threat or flee from it. In addition, they dilate the airways, raising blood oxygen levels. It also prompts vasodilation, further increasing the oxygenation of important organs such as the lungs, brain, heart, and skeletal muscle. At the same time, it triggers vasoconstriction to blood vessels serving less essential organs such as the gastrointestinal tract, kidneys, and skin and downregulates some components of the immune system. Other effects include a dry mouth, loss of appetite, pupil dilation, and a loss of peripheral vision. The major hormones of the adrenal glands and their effects are summarized in Table 9.3c.
Table 9.3c. Hormones of the Adrenal Glands[18]
Adrenal Gland | Hormones | Effect |
---|---|---|
Adrenal Cortex | Aldosterone | Increases blood Na+ levels, water reabsorption, and K+ excretion by kidneys |
Adrenal Cortex | Cortisol, corticosterone, and cortisone | Increases blood glucose levels during the stress response, reduces inflammation, and in high amounts suppresses immunity |
Adrenal Medulla | Epinephrine and norepinephrine | Stimulates fight-or-flight response |
Pineal Gland
The pineal gland is located inferior and somewhat posterior to the thalamus in the brain. It is a tiny endocrine gland whose functions are not entirely clear. The pineal gland is known to secrete the hormone melatonin, which is derived from serotonin.
The secretion of melatonin varies according to the level of light received from the environment. When light stimulates the retinas of the eyes, blood levels of melatonin fall, promoting wakefulness. In contrast, as light levels decline, such as during the evening, melatonin production increases, boosting blood levels and causing drowsiness.
The secretion of melatonin may influence the body’s circadian rhythms, the dark-light fluctuations that affect not only sleepiness and wakefulness, but also appetite and body temperature. Interestingly, children have higher melatonin levels than adults, which may prevent the release of gonadotropins from the anterior pituitary, thereby inhibiting the onset of puberty. Finally, an antioxidant role of melatonin is the subject of current research.
Jet lag occurs when a person travels across several time zones and feels sleepy during the day or wakeful at night. Traveling across multiple time zones significantly disturbs the light-dark cycle regulated by melatonin. It can take up to several days for melatonin synthesis to adjust to the light-dark patterns in the new environment, resulting in jet lag. Some air travelers take melatonin supplements to induce sleep.
See Figure 9.14[19] for an illustration of the pineal gland.
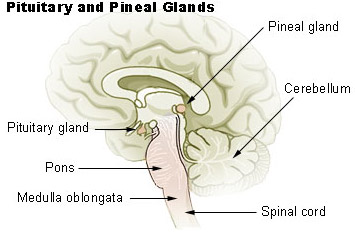
Ovaries and Testes
The ovaries in the female and the testes in the male are referred to as the gonads. Refer back to Figure 9.1 for an illustration of the ovaries and testes in the endocrine system. The gonads are responsible for producing secondary sex characteristics, as well as the reproductive cells – the sperm in males and the ova (eggs) in females.
The primary hormone produced by the testes is testosterone, a steroid hormone important in the development of the reproductive system, the maturing of sperm cells, and the development of secondary sex characteristics such as a deepened voice, body hair, and increased muscle mass. Interestingly, testosterone is also produced in the ovaries, but at a much-reduced level.
The primary hormones produced by the ovaries are estrogens, which include estradiol, estriol, and estrone. Estrogens play an important role in a larger number of physiological processes, including the development of the reproductive system, regulation of the menstrual cycle, the development of secondary sex characteristics such as increased adipose tissue and the development of breast tissue, and the maintenance of pregnancy.
Another significant ovarian hormone is progesterone, which contributes to regulation of the menstrual cycle and is important in preparing the body for pregnancy, as well as maintaining pregnancy.
Table 9.3d summarizes hormones produced by the testes and ovaries and their effects.
Table 9.3d. Reproductive Hormones and Their Effects[20]
Gonad | Hormones | Effect |
---|---|---|
Testes | Testosterone | Stimulates development of secondary sex characteristics and sperm production |
Ovaries | Estrogens and progesterone | Stimulates development of secondary sex characteristics, prepares the body for childbirth, and regulates the menstrual cycle |
Everyday Connection: Anabolic Steroids
The endocrine system can be exploited for illegal or unethical purposes. A prominent example of this is the use of steroid drugs by professional athletes. Commonly used for performance enhancement, anabolic steroids are synthetic versions of the sex hormone testosterone. By boosting natural levels of this hormone, athletes experience increased muscle mass. Synthetic versions of human growth hormone are also used to build muscle mass.
The use of performance-enhancing drugs is banned by all major collegiate and professional sports organizations in the United States because they impart an unfair advantage to athletes who take them. In addition, the drugs can cause significant and dangerous side effects. For example, anabolic steroid use can increase cholesterol levels, raise blood pressure, and damage the liver. Altered testosterone levels (both too low or too high) have been implicated in causing structural damage to the heart and increasing the risk for cardiac arrhythmias, heart attacks, congestive heart failure, and sudden death. Paradoxically, steroids can have effects such as shriveled testicles and enlarged breast tissue. In females, their use can cause effects such as an enlarged clitoris and growth of facial hair. In all people, their use can promote increased aggression (commonly known as “roid-rage”), depression, sleep disturbances, severe acne, and infertility.
Pancreas
The pancreas is a long, slender organ, most of which is located posterior to the bottom half of the stomach. See Figure 9.15[21] for an illustration of the pancreas. Although it is primarily an exocrine gland, secreting a variety of digestive enzymes, the pancreas also has an endocrine function. Its pancreatic islets—clusters of cells formerly known as the islets of Langerhans—secrete the hormones glucagon, insulin, somatostatin, and pancreatic polypeptide (PP).
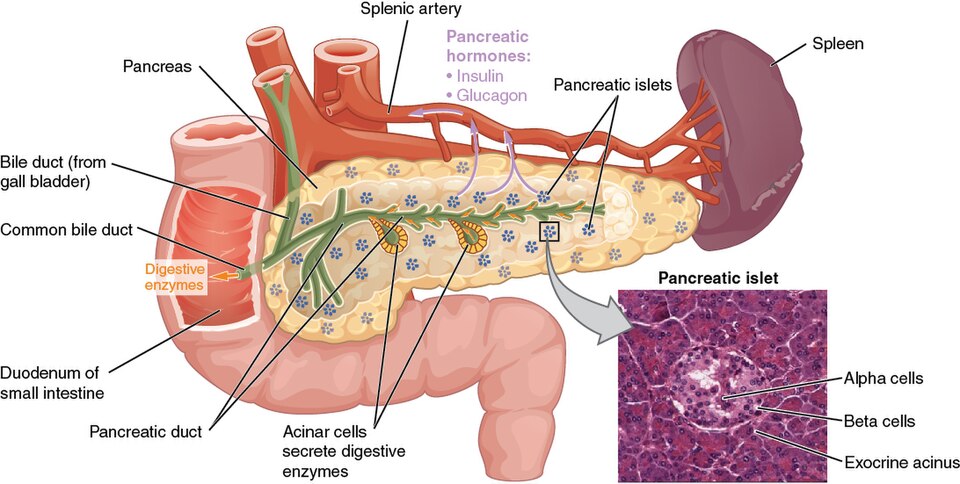
Cells and Secretions of the Pancreatic Islets
The pancreatic islets contain four types of cells:
- Alpha cells secrete the hormone glucagon, which increases blood glucose. Low blood glucose levels stimulate its release.
- Beta cells secrete the hormone insulin, which decreases blood glucose levels. Elevated blood glucose levels stimulate the release of insulin.
- Delta cells secrete the peptide hormone somatostatin. Somatostatin is also released by the hypothalamus (as GHIH), the stomach, and the intestines. Somatostatin inhibits the release of both glucagon and insulin.
- PP cells secrete pancreatic polypeptide hormone. It is thought to play a role in appetite, as well as in the regulation of pancreatic exocrine and endocrine secretions. Pancreatic polypeptide released following a meal may reduce further food consumption; however, it is also released in response to fasting.
Regulation of Blood Glucose Levels by Insulin and Glucagon
Glucose is the preferred fuel for all body cells. The body derives glucose from the breakdown of the carbohydrate-containing foods and drinks we consume. Glucose not immediately used by cells for fuel can be stored by the liver and muscles as glycogen or converted to triglycerides and stored in the adipose tissue. Hormones regulate both the storage and the utilization of glucose. Receptors located in the pancreas sense blood glucose levels, and subsequently, the pancreatic cells secrete glucagon or insulin to maintain normal levels.
Glucagon
Receptors in the pancreas can sense the decline in blood glucose levels, such as during periods of fasting or during prolonged labor or exercise. In response, the alpha cells of the pancreas secrete the hormone glucagon, which has several effects:
- It stimulates the liver to convert its stores of glycogen back into glucose. This response is known as glycogenolysis. The glucose is then released into circulation for use by body cells.
- It stimulates the liver to take up amino acids from the blood and convert them into glucose. This response is known as gluconeogenesis.
- It stimulates lipolysis, the breakdown of stored triglycerides into free fatty acids and glycerol. Some of the free glycerol released into the bloodstream travels to the liver, which converts it into glucose. This is also a form of gluconeogenesis.
Together, these actions increase blood glucose levels. The activity of glucagon is regulated through a negative feedback mechanism with rising blood glucose levels inhibiting further glucagon production and secretion. See Figure 9.16[22] for an illustration of the control of glucose levels.
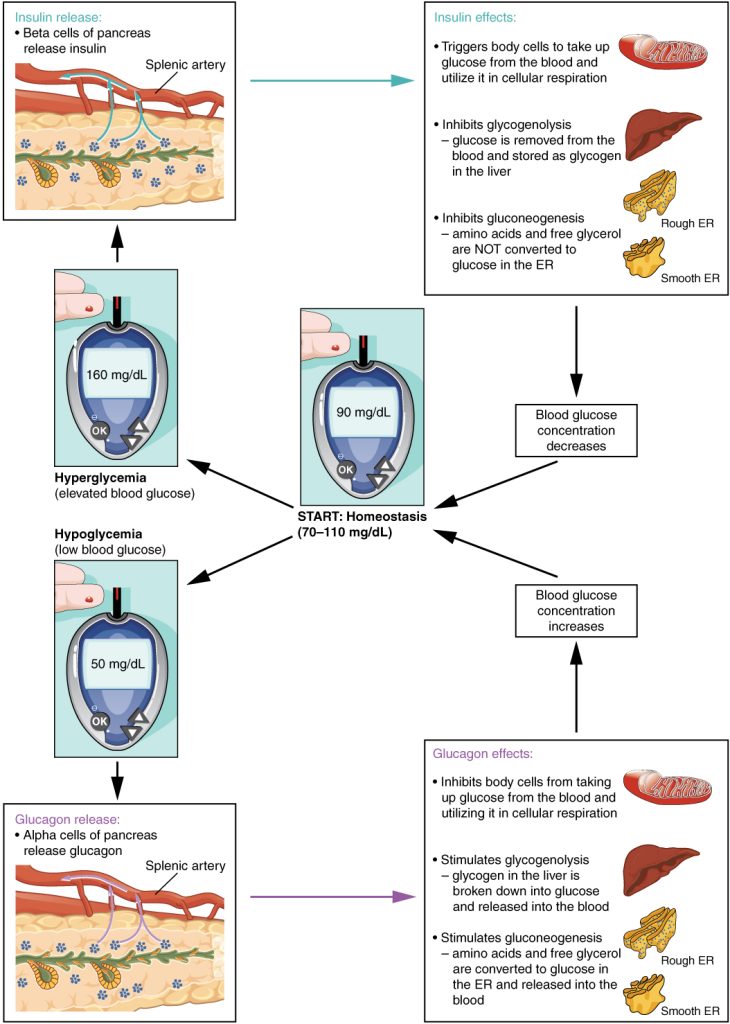
Insulin
The primary function of insulin is to facilitate the uptake of glucose into body cells, which subsequently lowers blood glucose levels Red blood cells, as well as cells of the brain, liver, kidneys, and the lining of the small intestine, do not have insulin receptors on their cell membranes and do not require insulin for glucose uptake. Although all other body cells do require insulin if they are to take glucose from the bloodstream, skeletal muscle cells and adipose cells are the primary targets of insulin.
Insulin also reduces blood glucose levels by stimulating glycolysis, the breakdown of glucose for generation of ATP. Moreover, it stimulates the liver to convert excess glucose into glycogen for storage, and it inhibits enzymes involved in glycogenolysis and gluconeogenesis. Finally, insulin promotes triglyceride and protein synthesis. The secretion of insulin is regulated through a negative feedback mechanism. As blood glucose levels decrease, further insulin release is inhibited. The pancreatic hormones and their effects are summarized in Table 9.3e.
Table 9.3e. Hormones of the Pancreas
Associated Hormones | Effect |
---|---|
Insulin (beta cells) | Decreases blood glucose levels |
Glucagon (alpha cells) | Increases blood glucose levels |
Somatostatin (delta cells) | Inhibits insulin and glucagon release |
Pancreatic polypeptide (PP cells) | Plays a role in appetite |
- Betts, J. G., Young, K. A., Wise, J. A., Johnson, E., Poe, B., Kruse, D. H., Korol, O., Johnson, J. E., Womble, M., & DeSaix, P. (2022). Anatomy and physiology 2e. OpenStax. https://openstax.org/books/anatomy-and-physiology-2e/pages/1-introduction ↵
- “eade2d286c1bf61900ea443ba642f097fc0c334d” by Rice University/Open Stax is licensed under CC BY 4.0. Access for free at https://openstax.org/books/anatomy-and-physiology-2e/pages/17-1-an-overview-of-the-endocrine-system#fig-ch18_01_01 ↵
- Betts, J. G., Young, K. A., Wise, J. A., Johnson, E., Poe, B., Kruse, D. H., Korol, O., Johnson, J. E., Womble, M., & DeSaix, P. (2022). Anatomy and physiology 2e. OpenStax. https://openstax.org/books/anatomy-and-physiology-2e/pages/1-introduction ↵
- “1805_Negative_Feedback_Loop” by OpenStax College is licensed under CC BY 3.0 ↵
- “1806_The_Hypothalamus-Pituitary_Complex” by OpenStax College is licensed under CC BY 3.0 ↵
- “1808_The_Anterior_Pituitary_Complex” by OpenStax College is licensed under CC BY 3.0 ↵
- “1809_Hormonal_Regulation_of_Growth” by OpenStax College is licensed under CC BY 3.0 ↵
- “Julius_Koch_Family_portrait.jpg” by unknown author is in the Public Domain. ↵
- “People_with_dwarfism_from_the_Philippines_in_1914,_"Philipino_midgets"_LCCN2007680836_(cropped)” by Gerhard Sisters is in the Public Domain. ↵
- “1810_Major_Pituitary_Hormones” by OpenStax College is licensed under CC BY 3.0 ↵
- “1811_The_Thyroid_Gland” by OpenStax College is licensed under CC BY 3.0 ↵
- “1813_A_Classic_Negative_Feedback_Loop” by OpenStax College is licensed under CC BY 3.0 ↵
- Cleveland Clinic. (2022). Thyroid hormone. https://my.clevelandclinic.org/health/articles/22391-thyroid-hormone ↵
- Betts, J. G., Young, K. A., Wise, J. A., Johnson, E., Poe, B., Kruse, D. H., Korol, O., Johnson, J. E., Womble, M., & DeSaix, P. (2022). Anatomy and physiology 2e. OpenStax. https://openstax.org/books/anatomy-and-physiology-2e/pages/1-introduction ↵
- “1814_The_Parathyroid_Glands” by OpenStax College is licensed under CC BY 3.0 ↵
- “1817_The_Role_of_Parathyroid_Hormone_in_Maintaining_Blood_Calcium_Homeostasis” by OpenStax College is licensed under CC BY 3.0 ↵
- “1818_The_Adrenal_Glands” by OpenStax College is licensed under CC BY 3.0 ↵
- Betts, J. G., Young, K. A., Wise, J. A., Johnson, E., Poe, B., Kruse, D. H., Korol, O., Johnson, J. E., Womble, M., & DeSaix, P. (2022). Anatomy and physiology 2e. OpenStax. https://openstax.org/books/anatomy-and-physiology-2e/pages/1-introduction ↵
- ”Illu_pituitary_pineal_glands” by unknown author, National Cancer Institute is in the Public Domain. ↵
- Betts, J. G., Young, K. A., Wise, J. A., Johnson, E., Poe, B., Kruse, D. H., Korol, O., Johnson, J. E., Womble, M., & DeSaix, P. (2022). Anatomy and physiology 2e. OpenStax. https://openstax.org/books/anatomy-and-physiology-2e/pages/1-introduction ↵
- “1820_The_Pancreas” by OpenStax College is licensed under CC BY 3.0 ↵
- “1822_The_Homostatic_Regulation_of_Blood_Glucose_Level” by OpenStax College is licensed under CC BY 3.0 ↵
Glands that secrete hormones directly into the bloodstream.
A cell that has specific receptors for a hormone and responds when the hormone binds to it.
Glands that release their secretions through ducts to the surface of an organ or tissue.
Also known as a sensor, monitors a physiological value, such as temperature, respiratory rate, blood pressure, etc.
Hormones derived from amino acids include amines, peptides, and proteins.
Hormones derived from lipids.
A protein located either inside the cell or within or on the cell membrane that receives a hormone’s message.
A process where target cells reduce the number of hormone receptors to decrease their response to high hormone levels.
A process where target cells increase the number of hormone receptors to increase their sensitivity to hormones present.
The presence of one hormone enables another hormone to act.
When two hormones with similar effects produce an amplified or bigger response together.
When two hormones have opposing effects.
Amplify or exaggerate the original stimulus.
The original stimulus is reduced or stopped.
The “command center” of the endocrine system.
A pea-sized organ suspended from a stem called the infundibulum (or pituitary stalk), also known as the hypophysis.
A thin connection between the hypothalamus and the pituitary gland that allows hormones and signals to pass between them.
Stimulates the secretion of hormones from the anterior pituitary gland.
Slows or stops the secretion of hormones from the anterior pituitary gland.
Also called somatotropin, a protein hormone from the anterior pituitary that supports growth by promoting protein synthesis and tissue building.
Hormones released by the liver and other tissues in response to growth hormone; they help promote cell growth and tissue repair.
A disorder in children that is caused by the secretion of abnormally large amounts of hGH, resulting in excessive growth.
A disorder in adults caused by excessive growth hormone that results in thickening of bones in the face, hands, and feet after normal growth has stopped.
A disorder where abnormally low levels of hGH in children can cause growth impairment.
Also called thyrotropin, a hormone from the anterior pituitary that signals the thyroid gland to release thyroid hormones.
Also called corticotropin, a hormone from the anterior pituitary that stimulates the adrenal cortex to release corticosteroid hormones like cortisol.
Hormones from the anterior pituitary that control the ovaries in females and testes in males, regulating reproductive functions.
A gonadotropin that promotes the production and development of sex cells (ova in females and sperm in males).
A gonadotropin that triggers ovulation in females and stimulates testosterone production in males.
Promotes lactation (milk production).
A hormone secreted between the pituitary lobes that stimulates melanin production in the skin in response to UV light exposure.
A hormone that stimulates uterine contractions and cervix dilation to help with childbirth.
The concentration of dissolved substances (solutes) in the blood, which can change due to diet, illness, medications, or other factors.
Specialized cells in the hypothalamus that detect changes in blood osmolality by sensing solute levels like sodium.
A hormone released by the posterior pituitary that helps the body retain water when blood osmolality is high, such as during dehydration.
A butterfly-shaped organ in the neck, located in front of the trachea and below the larynx.
A thyroid hormone with three iodine atoms that helps regulate metabolism.
A thyroid hormone with four iodine atoms that regulates metabolism and is converted into the active form, T3.
A hormone from the thyroid’s parafollicular (C) cells that lowers blood calcium levels when they rise too high.
Tiny, round structures usually found embedded in the posterior surface of the thyroid gland.
The major hormone responsible for increasing blood calcium levels.
The active form of vitamin D3 produced in the kidneys under the influence of parathyroid hormone (PTH); it helps the intestines absorb more calcium from food.
Wedges of tissue attached to the top of each kidney that produce various hormones.
The outer layer of the adrenal glands that produces steroid hormones and is part of the hypothalamic-pituitary-adrenal (HPA) axis.
Neuroendocrine tissue that is an extension of the autonomic nervous system.
Hormones produced by the adrenal cortex that regulate minerals like sodium and potassium in the body.
The main mineralocorticoid hormone that helps balance sodium and potassium levels and controls blood pressure and volume.
Hormones from the adrenal cortex that help regulate glucose (sugar) metabolism and the body’s response to stress.
The main glucocorticoid hormone that helps the body respond to stress by breaking down stored nutrients for energy and slowing tissue building.
A hormone from the adrenal medulla that is produced in large amounts and helps the body respond quickly to stress. Also called Adrenaline.
A hormone from the adrenal medulla that works with epinephrine to prepare the body for stress but is produced in smaller amounts. Also called Noradrenaline
A small gland in the brain below and behind the thalamus that produces melatonin, a hormone involved in regulating sleep.
A hormone produced by the pineal gland that helps regulate the sleep-wake cycle.
The main hormone produced by the testes that supports sperm development and causes male secondary sex traits like a deeper voice and muscle growth.
Hormones produced by the ovaries, including estradiol, estriol, and estrone, that regulate the reproductive system, menstrual cycle, female secondary sex traits, and pregnancy.
A hormone from the ovaries that helps regulate the menstrual cycle and supports pregnancy preparation and maintenance.
A long, thin organ located mostly behind the lower part of the stomach that produces hormones and digestive enzymes.
Clusters of cells in the pancreas that produce hormones like glucagon, insulin, somatostatin, and pancreatic polypeptide.
Secrete the hormone glucagon, which increases blood glucose. Low blood glucose levels stimulate its release.
Secrete the hormone insulin, which decreases blood glucose levels. Elevated blood glucose levels stimulate the release of insulin.
A hormone secreted by beta cells of the pancreas that decreases blood glucose levels. Elevated blood glucose levels stimulate its release.
Secrete the peptide hormone somatostatin. Somatostatin is also released by the hypothalamus (as GHIH), the stomach, and the intestines. It inhibits the release of both glucagon and insulin.
Secrete pancreatic polypeptide hormone. It is thought to play a role in appetite and the regulation of pancreatic exocrine and endocrine secretions.
The storage form of glucose in humans and other animals and is made up of monomers (building blocks) of glucose bonded together into long chains.
A hormone secreted by alpha cells of the pancreas in response to low blood glucose levels. It raises blood glucose by triggering the release of stored glucose from the liver.
The process of converting stored glycogen in the liver back into glucose, which is then released into the blood.
The process by which the liver converts amino acids from the blood into glucose.
The process of breaking down glucose to produce ATP (energy), stimulated by insulin to help lower blood glucose levels.