7.3 Skeletal Muscle
Skeletal Muscle[1]
Skeletal muscle is attached to bones, so its contraction makes movement, facial expressions, posture, and other voluntary movements of the body possible. Forty percent of your body mass is made up of skeletal muscle.
Skeletal Muscle Organization
Each skeletal muscle is an organ that consists of various integrated tissues. These tissues include the skeletal muscle fibers (muscle cells or myocytes), blood vessels, nerve fibers, and connective tissue. Every skeletal muscle is richly supplied by blood vessels for nourishment, oxygen delivery, and waste removal. Inside each skeletal muscle, muscle fibers are organized into individual bundles or groups of fibers called fascicles.
Each skeletal muscle has three layers of connective tissue (called “mysia”) that enclose it, provide structure to the muscle as a whole, and also bundle the muscle fibers within the muscle. See Figure 7.2[2] for an illustration of the structure of skeletal muscle tissue. The three layers of connective tissue are the epimysium, the perimysium and the endomysium:
- Epimysium. The epimysium is a sheath of dense, irregular connective tissue that wraps around the outside of the entire muscle. It allows the muscle to contract and move powerfully while maintaining its structure. The epimysium separates muscle from other tissues and organs in the area, allowing the muscle to move independently.
- Perimysium. The perimysium is a layer of connective tissue that surrounds each fascicle (bundle of muscle fibers).
- Endomysium. The endomysium is a thin connective tissue layer of collagen and reticular fibers covering each individual muscle fiber. The endomysium contains the extracellular fluid and nutrients to support the muscle fiber. These nutrients are supplied via blood to the muscle tissue.
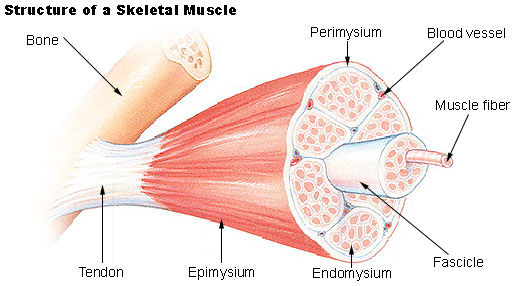
View a supplementary video[3] on muscle structure: The Structure of the Muscle Organ.
To move the skeleton, the tension created by the contraction of the fibers in most skeletal muscles is transferred to tendons. Tendons are strong bands of dense, regular connective tissue that connect muscles to bones. This bone connection is why this muscle tissue is called skeletal muscle.
The collagen of the three mysia layers (epimysium, perimysium and endomysium) intertwine with the collagen of the tendon, and the other end of the tendon is connected to the periosteum (outer covering) of the bone. The tension created by contraction of the muscle fibers is then transferred through the mysia, to the tendon, and then to the periosteum to pull on the bone for movement of the skeleton. In a way, your body is like a sophisticated marionette inside. Skeletal muscles contract and pull on tendons, which then pull on bones to create movement.
In other places, the mysia may fuse with a broad, flat tendon-like sheet called an aponeurosis, or to fascia, the connective tissue between skin and bones. The broad sheet of connective tissue in the lower back that the latissimus dorsi muscles (the “lats”) fuse into is an example of an aponeurosis.
Skeletal Muscle Fibers
Skeletal muscle cells are long and cylindrical and are commonly referred to as muscle fibers. Skeletal muscle fibers can be quite large for human cells, with diameters up to 100 μm and lengths up to 30 cm (11.8 in) in the sartorius muscle of the upper leg. Muscle fibers have up to 100 nuclei in each fiber. Multiple nuclei mean multiple copies of genes, which allows the production of large amounts of proteins and enzymes needed for muscle contraction.
The plasma membrane of a muscle fiber is called the sarcolemma. The cytoplasm is referred to as sarcoplasm, and the specialized smooth endoplasmic reticulum, which stores, releases, and retrieves calcium ions (Ca2+), is called the sarcoplasmic reticulum (SR).
Inside the muscle fiber are myofibrils, long, cylindrical organelles that run parallel within the muscle fiber and contain the sarcomeres, the basic unit of muscle contraction. See Figure 7.3[4] for an illustration of the organization of a skeletal muscle fiber.
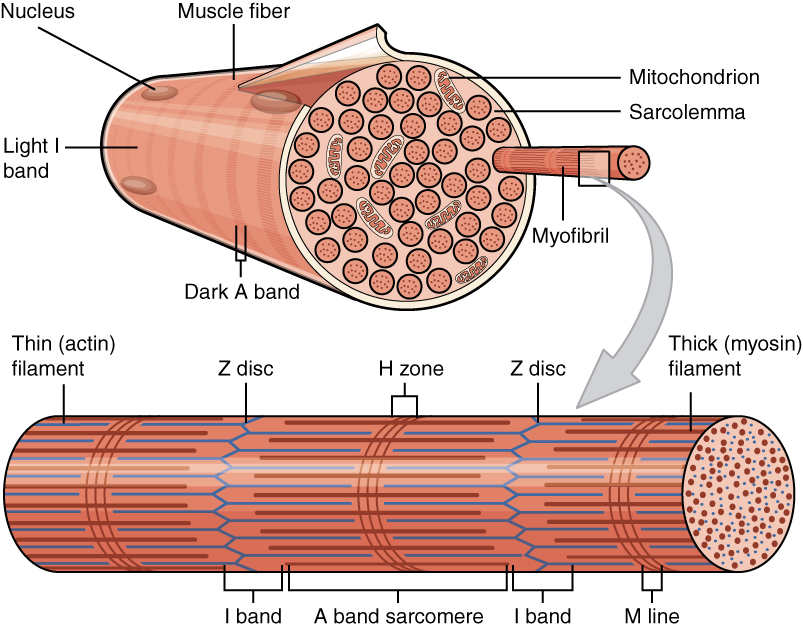
Sarcomere
The smallest functioning or basic unit of a skeletal muscle fiber is the sarcomere, a highly organized arrangement of the contractile proteins actin (thin filament) and myosin (thick filament), along with other regulatory and support proteins. The arrangement of actin and myosin in the sarcoplasm of individual muscle fibers creates a pattern, or stripes, called striations when viewed under a microscope.
The sarcomere is bundled within the myofibril that runs the entire length of the muscle fiber and attaches to the sarcolemma at its end. As myofibrils contract, the entire muscle cell contracts. Because myofibrils are only approximately 1.2 μm in diameter, hundreds to thousands (each with thousands of sarcomeres) can be found inside one muscle fiber. Each sarcomere is approximately 2 μm in length and is bordered by structures called Z-discs (also called Z-lines) to which the actin myofilaments are anchored. Because the actin forms strands that are thinner than the myosin, they are called the thin filaments of the sarcomere. Likewise, because the myosin strands have more mass and are thicker, they are called the thick filaments of the sarcomere. See Figure 7.4[5] for an illustration of a sarcomere and Figure 7.5[6] for a microscopic image of a sarcomere.
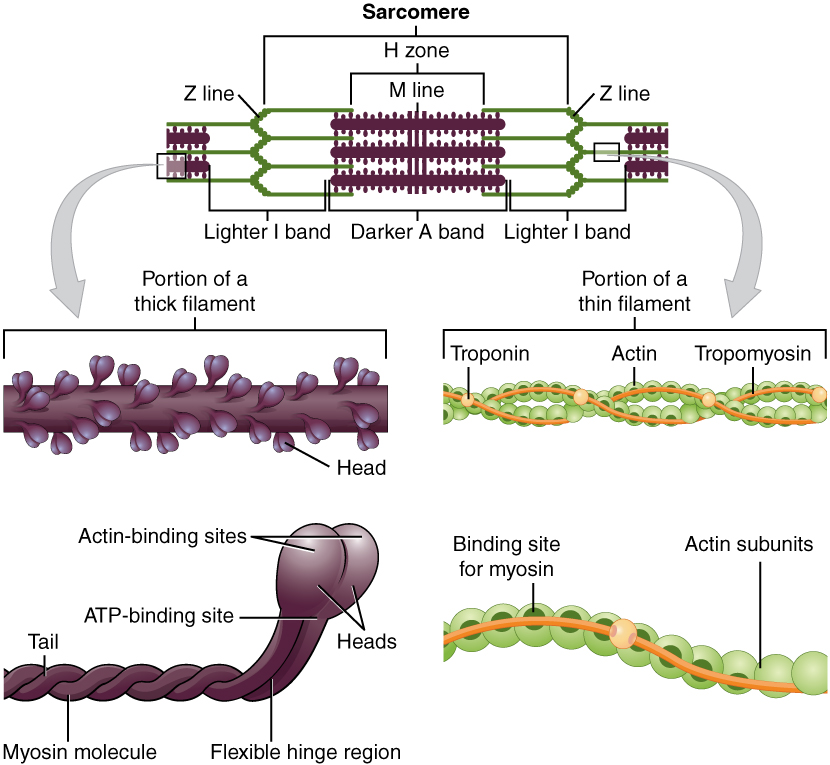
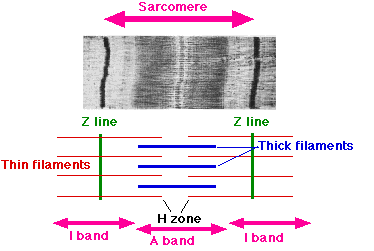
Skeletal Muscle Contraction
Motor Units
Every skeletal muscle fiber must be innervated by a motor neuron in order to contract. One motor neuron and the number of fibers it controls is called a motor unit. The size of a motor unit varies depending on the nature of the muscle.
A small motor unit is an arrangement where a single motor neuron controls a small number of muscle fibers in a muscle. Small motor units allow very fine motor control of the muscle. The best example in humans is the small motor units of the extraocular eye muscles that move the eyeballs. There are thousands of muscle fibers in each muscle, but every six or so fibers are stimulated by a single motor neuron. This allows for precise control of eye movements so that both eyes can quickly focus on the same object. Small motor units are also involved in the many fine motor movements of the fingers and thumb of the hand for grasping, texting, etc.
A large motor unit is an arrangement where a single motor neuron controls a large number of muscle fibers in a muscle. Large motor units are concerned with simple, or “gross,” movements, such as powerfully extending the knee joint. The best example is the large motor units of the thigh muscles or back muscles, where a single motor neuron will supply thousands of muscle fibers in a muscle.
There is a wide range of motor units within many skeletal muscles, which gives the nervous system a wide range of control over the muscle. The small motor units in the muscle will have smaller motor neurons that are more excitable, firing first to their skeletal muscle fibers, which also tend to be the smallest. Activation of these smaller motor units results in a relatively small degree of contractile strength (tension) generated in the muscle. As more strength is needed, larger motor units with bigger motor neurons are enlisted to activate larger muscle fibers. This increase in the number of active motor units produces an increase in muscle contraction known as recruitment. As more motor units are recruited, the muscle contraction grows progressively stronger. In some muscles, the largest motor units may generate a contractile force of 50 times more than the smallest motor units in the muscle. This allows a feather to be picked up using the biceps brachii arm muscle with minimal force, and a heavy weight to be lifted by the same muscle by recruiting the largest motor units.
When necessary, the maximal number of motor units in a muscle can be recruited simultaneously, producing the maximum force of contraction for that muscle, but this cannot last for very long because of the energy requirements to sustain the contraction. To prevent complete muscle fatigue, motor units are generally not all simultaneously active. Instead, some motor units rest while others are active, which allows for longer muscle contractions.
See Figure 7.6[7] for an illustration of motor units.
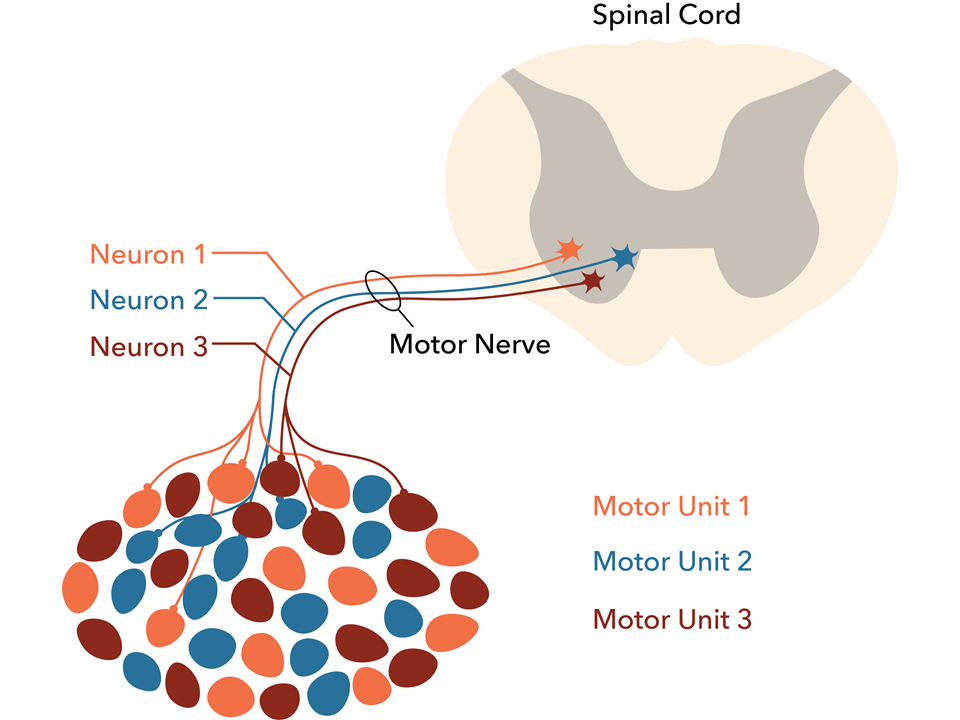
The Neuromuscular Junction
The neuromuscular junction (NMJ) is the region where a neuron meets and communicates with a skeletal muscle fiber. The two structures are very close together but don’t physically touch. There is a small gap of space between them called a synapse. The neuron will release many molecules of a neurotransmitter or chemical called acetylcholine that will stimulate the skeletal muscle to contract. This is where the muscle fiber first responds to signaling by the motor neuron. Every skeletal muscle fiber in every skeletal muscle is innervated by a motor neuron at the NMJ. Excitation signals from the neuron are the only way to activate the muscle fiber to contract.
Excitation-Contraction Coupling
All living cells have membrane potentials, or electrical gradients across their cell membranes. Additional information about the electrical activity of excitable cells is discussed in the “Nervous System” chapter. The inside of the membrane is usually around -60 to -90 mV compared to the outside of the cell. This is referred to as a cell’s membrane potential. Neurons and muscle cells can use their membrane potentials to generate electrical signals. They do this by controlling the movement of charged particles, called ions, across their membranes to create electrical currents. This is achieved by opening and closing specialized protein channels in the membrane called ion channels.
The term excitation-contraction coupling refers to what must happen for a skeletal muscle fiber to contract. First, the sarcolemma must be “excited”— it must be stimulated to create an action potential. The muscle fiber action potential travels along the sarcolemma and is “coupled” to the actual contraction of the muscle fiber.
The brain initiates skeletal muscle contraction. To do so, it sends a signal down the spinal cord. From there, a motor neuron “tells” the muscle to contract. These neurons have long axons that transmit action potentials (electrical signals) all the way from the spinal cord to the muscle itself (which may be up to three feet away).
Signaling begins when an action potential travels along the axon of a motor neuron to the neuromuscular junction (NMJ). At the NMJ, the motor axon terminal releases a neurotransmitter (a chemical messenger) called acetylcholine (ACh). The ACh molecules diffuse across the space between the motor neuron and the sarcolemma called the synaptic cleft and bind to ACh receptors located on the other side of the synapse. The ACh receptors are found on the motor end plate of the sarcolemma. See Figure 7.7[8] for an illustration of the neuromuscular junction and Figure 7.8[9] for a microscopic image of a neuromuscular junction.
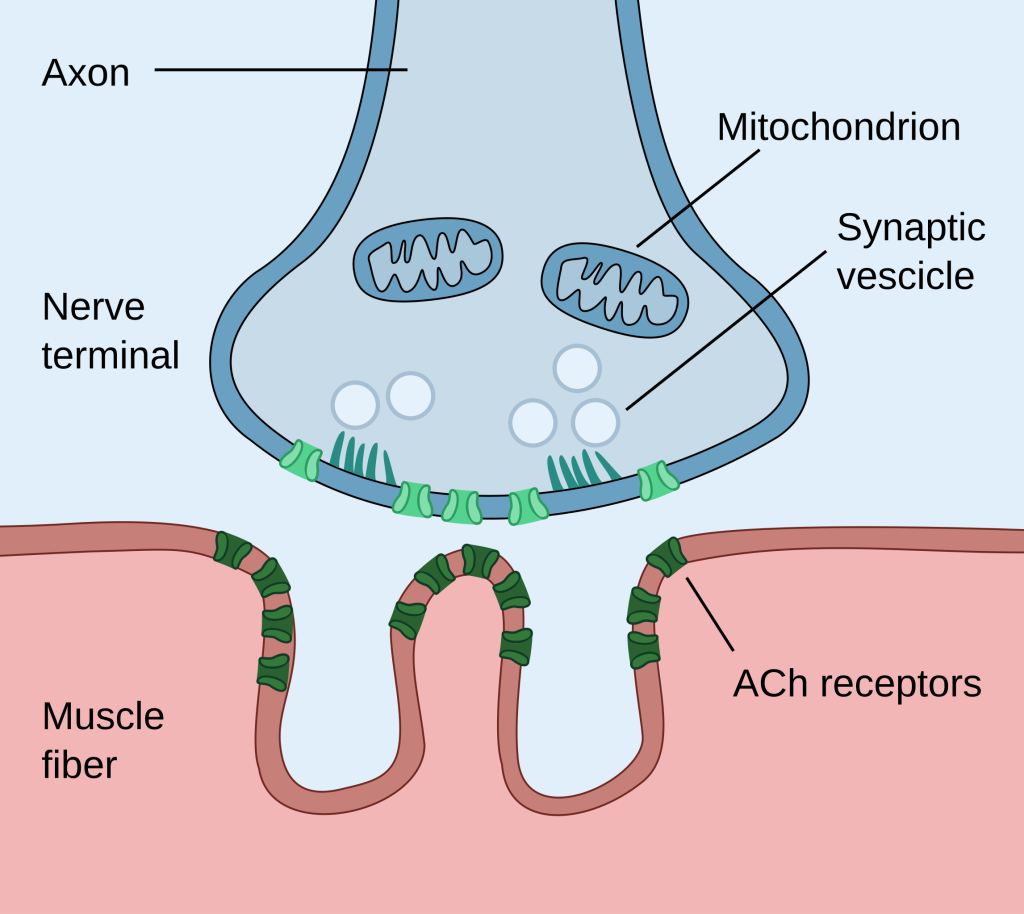
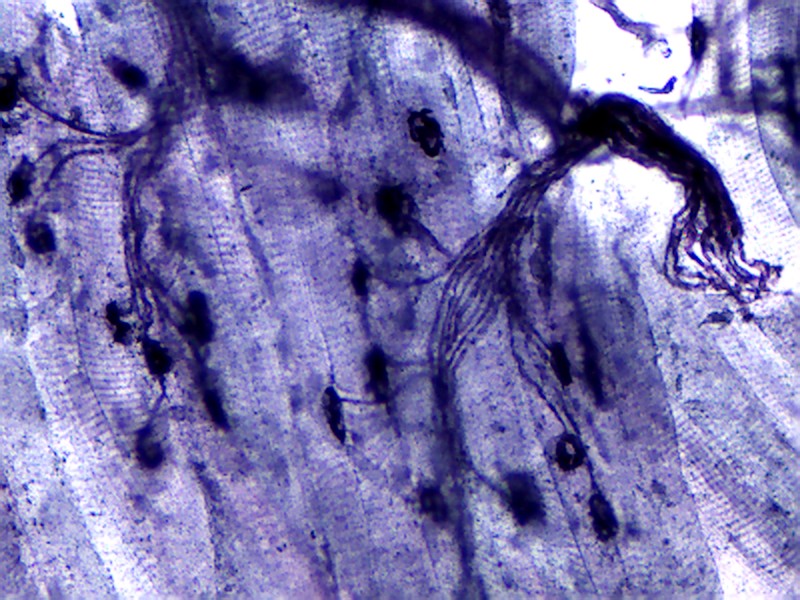
Once ACh binds to the ACh receptors on the motor end plate, a channel opens and positively charged sodium ions pass through into the muscle fiber. This causes the muscle fiber to depolarize, meaning that the membrane potential inside the muscle fiber becomes less negative. As the membrane depolarizes, another set of ion channels called voltage-gated sodium channels are triggered to open. Sodium ions enter the muscle fiber, and an action potential rapidly spreads (or “fires”) along the entire membrane to initiate excitation-contraction coupling. See Figure 7.9[10] for an illustration of the steps in muscle fiber contraction.
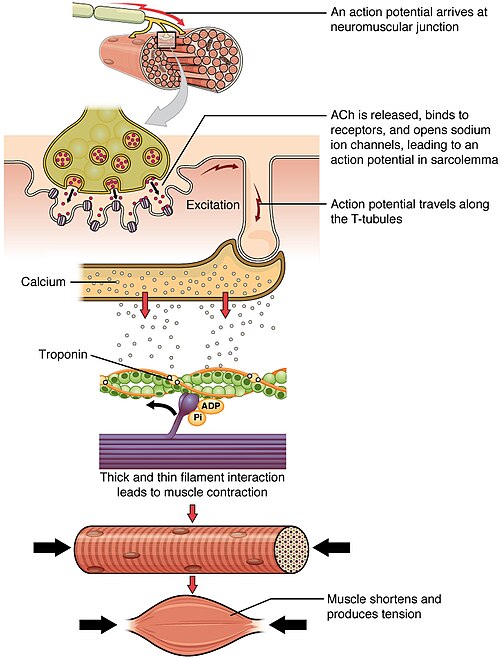
Things happen very quickly in the world of excitable membranes (just think about how quickly you can snap your fingers as soon as you decide to do it). Immediately following depolarization of the membrane, it repolarizes. In repolarization, voltage-gated potassium channels open and potassium ions leave the cell, re-establishing the negative membrane potential. Muscle contraction usually stops when signaling from the motor neuron ends. A muscle also will stop contracting when it runs out of ATP and becomes fatigued.
The Sliding Filament Model of Contraction
When signaled by a motor neuron, a skeletal muscle fiber contracts as the thin filaments (actin) are pulled by and then slide past the thick filaments (myosin) inside the sarcomeres. This process is known as the sliding filament model of muscle contraction. See Figure 7.10[11] for an illustration of the sliding filament of muscle contraction.
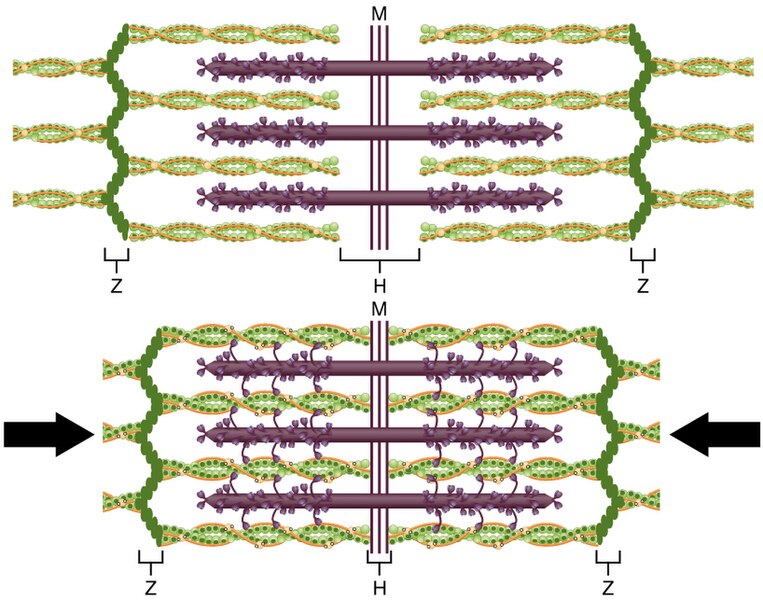
Muscle contraction is regulated by proteins and calcium ions. The actin filaments contain myosin-binding sites. These binding sites are the only places that the myosin heads can attach to the actin to pull them during contraction. When a muscle is relaxed, these binding sites are covered by a ribbon-like protein called tropomyosin that winds around the actin filaments. Another regulatory protein called troponin is attached to tropomyosin. For a muscle to contract, these binding sites have to be uncovered.
When a muscle has an action potential, calcium ions are released from the sarcoplasmic reticulum inside the muscle fiber where they are stored. Calcium ions then attach to the troponin molecules and change its shape, much like a key unlocking a lock. This allows the myosin heads to attach to actin. The thin filaments are then pulled by the myosin heads and slide toward the center of the sarcomere.
View these supplementary YouTube videos[12],[13] on the following topics:
ATP and Muscle Contraction
A huge amount of ATP is needed to power the muscle contraction cycle and keep skeletal muscles working. In fact, it is the loss of ATP that results in the rigor mortis observed soon after death. With no further ATP production possible, myosin cannot detach from actin, causing the rigidity seen in skeletal muscles.
Relaxation of a Skeletal Muscle
Relaxing skeletal muscle fibers, and ultimately, the skeletal muscle, begins with the motor neuron, which stops releasing its chemical signal, ACh, into the synapse at the NMJ. Because the skeletal muscle fiber has now “lost” its signal to contract, it no longer generates an action potential, and calcium ions are pumped back into the sarcoplasmic reticulum. When calcium ions are removed from troponin, the troponin-tropomyosin complex recovers the binding sites on actin so that myosin can no longer “grab” and slide it. As a result, the muscle relaxes.
Muscle Strength
The number of skeletal muscle fibers in a given muscle is genetically determined and does not change. Muscle strength is directly related to the number of myofibrils and sarcomeres within each fiber. Factors, such as hormones and stress (and artificial anabolic steroids), acting on the muscle can increase the production of sarcomeres and myofibrils within the muscle fibers, a change called hypertrophy, which results in the increased mass and bulk in a skeletal muscle. Likewise, decreased use of a skeletal muscle results in atrophy, where the number of sarcomeres and myofibrils disappear (but not the number of muscle fibers). It is common for a broken limb in a cast to show atrophied muscles when the cast is removed. This effect is temporary, and the muscle regains strength when the limb is used again. Certain diseases, such as polio, also lead to atrophied muscles.
To move an object, referred to as load, the sarcomeres in the muscle fibers of the skeletal muscle must shorten. The force generated by the contraction of the muscle (or shortening of the sarcomeres) is called muscle tension. Muscle tension is also generated when the muscle is contracting against a load that does not move, resulting in two main types of skeletal muscle contractions: isotonic contractions and isometric contractions. All these muscle activities are under the control of the nervous system.
Isotonic and Isometric Contractions
In isotonic contractions, the tension in the muscle stays constant, and movement occurs as the length of the muscle changes (shortens). There are two types of isotonic contractions: concentric and eccentric. A concentric contraction involves the muscle shortening to move a load. An example of this is the biceps brachii muscle contracting to bend the elbow when a hand weight is brought upward with increasing muscle tension. As the biceps brachii contracts, the angle of the elbow joint decreases as the forearm is brought toward the body. An eccentric contraction occurs as the muscle tension diminishes and the muscle lengthens. In this case, the hand weight is lowered in a slow and controlled manner. The tension is released from the biceps brachii and the angle of the elbow joint increases.
An isometric contraction occurs as the muscle produces tension without producing movement. For example, if one attempts to lift a hand weight that is too heavy, there will be sarcomere activation and shortening to a point, and ever-increasing muscle tension, but no change in the angle of the elbow joint. In everyday living, isometric contractions are active in maintaining posture and bone and joint stability. However, holding your head in an upright position occurs not because the muscles cannot move the head, but because the goal is to remain stationary and not produce movement. Most actions of the body are the result of a combination of isotonic and isometric contractions working together. See Figure 7.11[14] for an illustration of the isometric and isotonic contractions.
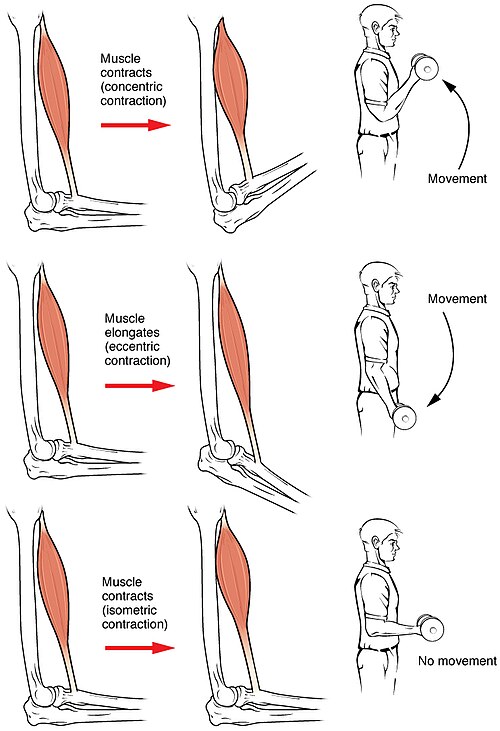
Muscle Tone
Skeletal muscles are rarely completely relaxed (flaccid). Even if a muscle is not producing movement, it contracts a small amount to produce muscle tone. The tension produced by muscle tone allows muscles to stabilize joints and maintain posture. Muscle tone is accomplished by a complex interaction between the nervous system and skeletal muscles that results in the activation of a few motor units at a time. In this manner, muscles never fatigue completely, as some motor units can recover while others are active.
Hypotonia
The absence of the low-level contractions that lead to muscle tone is referred to as hypotonia and can result from damage to parts of the brain, such as the cerebellum, the spinal cord, or from loss of innervation to a skeletal muscle, as in poliomyelitis. Hypotonic muscles have a flaccid (limp) appearance and display functional impairments, such as weak reflexes.
Hypertonia
Excessive muscle tone is referred to as hypertonia, accompanied by hyperreflexia (excessive reflex responses), and it is often the result of damage to upper motor neurons in the brain or spinal cord. Hypertonia can present with muscle rigidity (as seen in Parkinson’s disease) or spasticity, an increase in muscle tone and stiffness.
Performance-Enhancing Substances
Some athletes attempt to boost their performance by using various agents that may enhance muscle performance. Anabolic steroids are a well-known method to boost muscle mass and increase power output. Anabolic steroids are a form of testosterone, a male sex hormone that stimulates muscle formation, leading to increased muscle mass.
Endurance athletes may also try to boost the availability of oxygen to muscles by using substances such as erythropoietin (EPO), a hormone normally produced by the kidneys, which triggers the production of red blood cells. The extra oxygen carried by these blood cells can then be used by muscles. Human growth hormone (hGH) is another supplement, and although it can facilitate building muscle mass, its main role is to promote the healing of muscle and other tissues after strenuous exercise. Increased hGH may allow for faster recovery after muscle damage, reducing the rest required after exercise, and allowing for more sustained high-level performance.
Although performance-enhancing substances often do improve performance, most are banned by governing bodies in sports and are illegal for nonmedical purposes. Their use to enhance performance raises ethical issues of cheating because they give users an unfair advantage over nonusers. A greater concern, however, is that their use carries serious health risks. The side effects of these substances are often significant, irreversible, and in some cases fatal. The physiological strain caused by these substances is often greater than what the body can handle, leading to effects that are unpredictable and dangerous. Anabolic steroid use has been linked to infertility, aggressive behavior, cardiovascular disease, and brain cancer.
Similarly, some athletes have used creatine to increase power output. Creatine phosphate provides quick bursts of ATP to muscles in the initial stages of contraction. Increasing the amount of creatine available to cells is thought to produce more ATP and, therefore, increase explosive power output, although its effectiveness as a supplement has been questioned.
Interactions of Skeletal Muscles in the Body
Muscles are usually connected to bones by tendons. Some muscles are connected by an aponeurosis instead. Muscles have at least two attachment points. To pull on a bone and move the skeleton, a skeletal muscle must also be attached to a fixed part of the skeleton. The moveable end of a muscle that attaches to the bone being pulled is called the muscle’s insertion, and the end of the muscle attached to a fixed (stabilized) bone is called the origin.
Although a number of muscles may be involved in an action, the primary muscle involved is called the prime mover, or agonist. To lift a cup, a muscle called the biceps brachii is the prime mover; however, because it is assisted by the brachialis, the brachialis is called a synergist. A synergist can also be a fixator that stabilizes the bone attached to the prime mover’s origin. See Figure 7.12[15] for an illustration of prime movers and synergists.
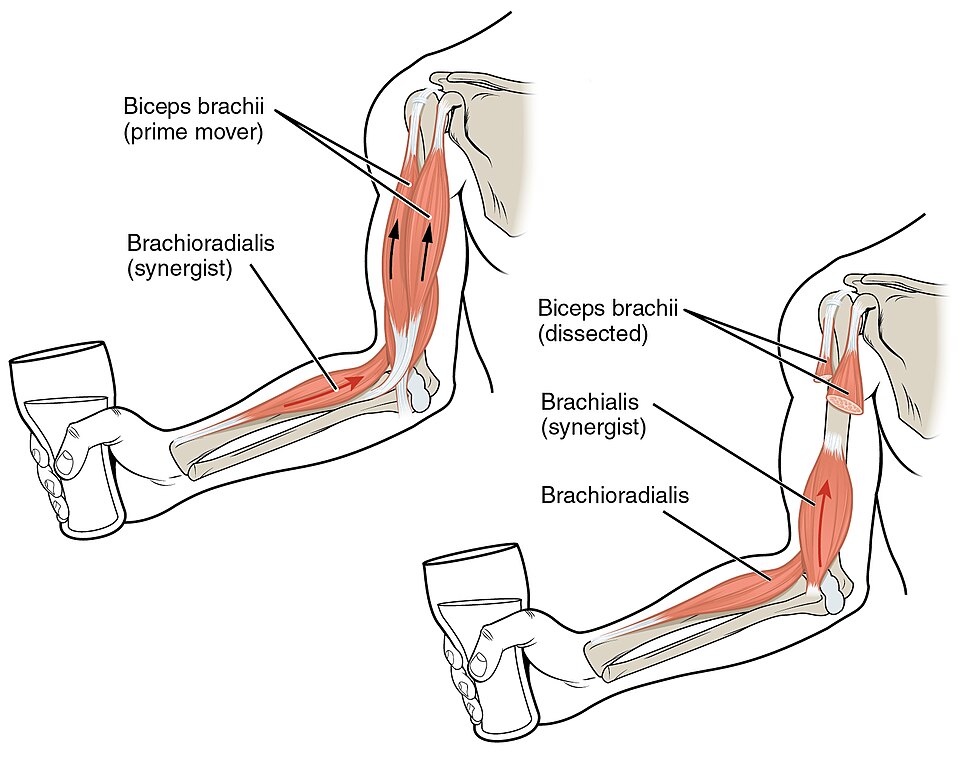
A muscle with the opposite action of the prime mover is called an antagonist. Antagonists play two important roles in muscle function: (1) they maintain body or limb position, such as holding the arm out or standing erect; and (2) they control rapid movement, as in shadow boxing without landing a punch or the ability to check the motion of a limb.
For example, to extend the knee, a group of four muscles called the quadriceps femoris in the anterior compartment of the thigh is activated (and would be called the agonists of knee extension). However, to flex the knee joint, an opposite or antagonistic set of muscles called the hamstrings is activated.
As you can see, these terms would also be reversed for the opposing action. If you consider the first action as the knee bending, the hamstrings would be called the agonists and the quadriceps femoris would then be called the antagonists. See Table 7.3a for an example of some agonists and antagonists.
Table 7.3a. Examples of Agonist and Antagonist Skeletal Muscle Pairs[16]
Agonist |
Antagonist |
Action(s) |
---|---|---|
Biceps brachii: anterior upper arm | Triceps brachii: posterior upper arm | The biceps brachii flexes the forearm, whereas the triceps brachii extends it. |
Hamstrings: group of three muscles in the posterior thigh | Quadriceps femoris: group of four muscles in the anterior thigh | The hamstrings flex the lower leg, whereas the quadriceps femoris extend it. |
There are also skeletal muscles that do not pull against the skeleton for movements. For example, there are the muscles that produce facial expressions. The insertions and origins of facial muscles are in the skin, allowing muscles to contract to form a smile or frown, form sounds or words, and raise the eyebrows. There also are skeletal muscles in the tongue and the external urinary and anal sphincters that allow for voluntary control of urination and defecation. In addition, the diaphragm contracts and relaxes to change the volume of the thoracic cavity during breathing, but it does not move the skeleton to do this.
Exercise and Stretching
When exercising, it is important to first warm up the muscles. Stretching pulls on the muscle fibers and results in an increased blood flow to the muscles being worked. Without a proper warm-up, it is possible to damage some of the muscle fibers or pull a tendon. A pulled tendon, regardless of location, results in pain, swelling, and diminished function.
Most of the joints used during exercise are synovial joints, which have synovial fluid in the joint space between two bones. Exercise and stretching may have a beneficial effect on synovial joints. Synovial fluid is a thin, but viscous film with the consistency of egg whites. After proper stretching and warm-up, the synovial fluid may become less viscous, allowing for better joint function.
Skeletal Muscle Actions
There are many types of actions that are caused by the contraction of muscles, such as flexion, extension, abduction, adduction, rotation, dorsiflexion, plantar flexion, supination, and pronation. These muscle actions are summarized in Table 7.3b.
Table 7.3b. Muscle Actions
Action |
Description |
---|---|
Flexion | Movement that decreases the angle between two bones, such as bending the arm at the elbow. |
Extension | Movement that increases the angle between two bones, such as straightening the arm at the elbow. |
Abduction | Movement of a limb away from the midline of the body. |
Adduction | Movement of a limb toward the midline of the body. |
Rotation | Circular movement around a central point. Internal rotation is toward the center of the body, and external rotation is away from the center of the body. |
Dorsiflexion | Decreasing the angle between the foot and the lower leg at the ankle (i.e., the foot moves upward towards the shin). |
Plantar Flexion | Increasing the angle between the foot and the lower leg at the ankle (i.e., the foot moves downward toward the ground, such as when pressing down on a gas pedal in a car). |
Supination | Movement of the hand or foot turning upward. When applied to the hand, it is the act of turning the palm upwards. When applied to the foot, it is the outward roll of the foot/ankle during normal movement. |
Pronation | Movement of the hand or foot turning downward. When applied to the hand, it is the act of turning the palm downward. When applied to the foot, it is the inward roll of the foot/ankle during normal movement. |
Eversion | Excessive movement turning the sole of the foot away from the midline of the body; a common cause of an ankle sprain. |
Inversion | Excessive movement turning the sole of the foot towards the midline; a common cause of an ankle sprain. |
See Figures 7.13[17] and 7.14[18] for illustrations of muscle actions.
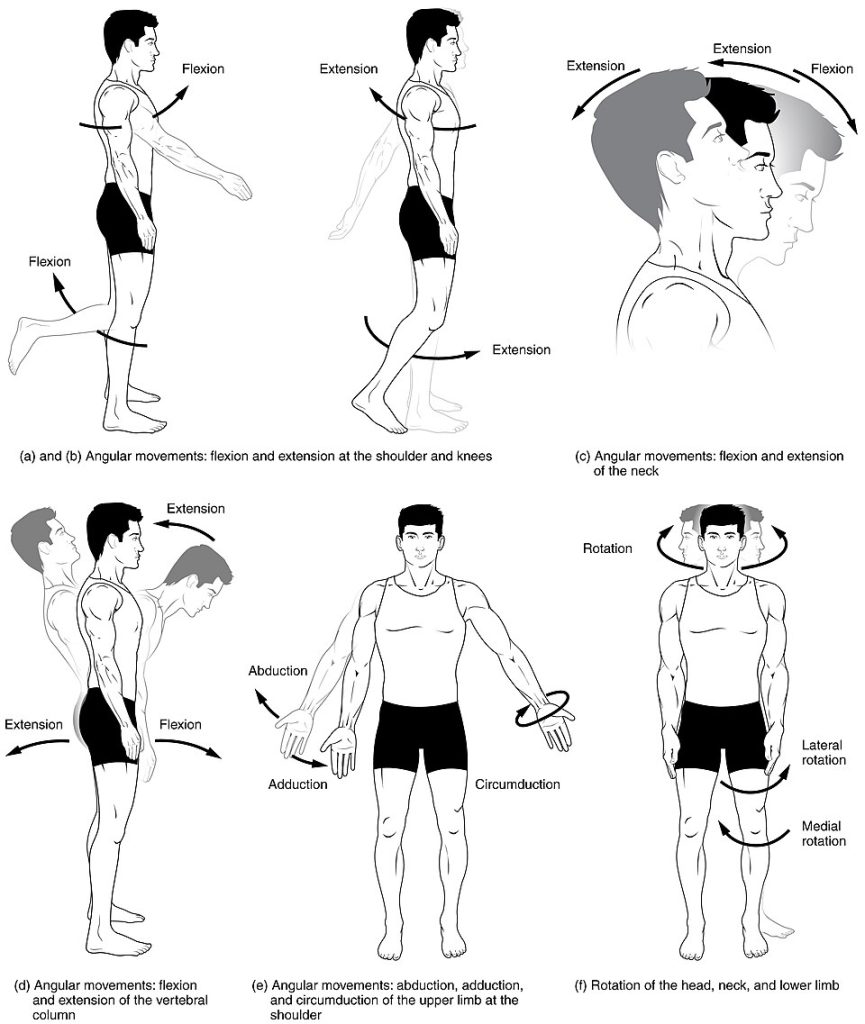
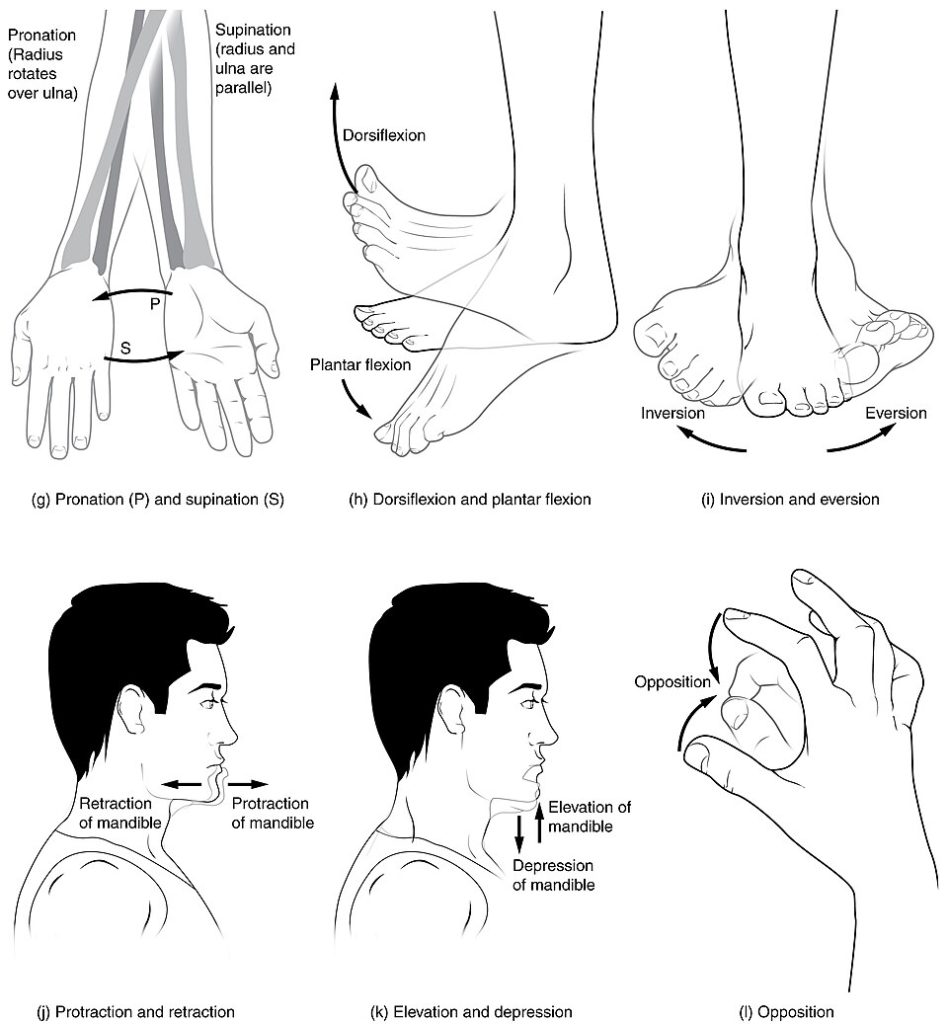
Patterns of Fascicle Organization
As previously discussed in this chapter, skeletal muscle is surrounded by connective tissue at three levels. Each muscle fiber (cell) is covered by endomysium, and the entire muscle is covered by epimysium. Groups of muscle fibers are “bundled” into fascicles and covered by connective tissue called perimysium. Fascicle arrangement by perimysium is correlated to the force generated by a muscle; it also affects the range of motion of the muscle. Based on the patterns of fascicle arrangement, skeletal muscles can be classified in several ways. The following are the most common fascicle arrangements.
Parallel muscles have fascicles that are arranged in the same direction as the long axis of the muscle. The majority of skeletal muscles in the body have this type of organization. Some parallel muscles are flat sheets that expand at the ends to make broad attachments. Other parallel muscles are rounder with tendons at one or both ends. Muscles that seem to be plump have a large mass of tissue located in the middle of the muscle, between the insertion and the origin, which is known as the central body. A more common name for this is belly. When a muscle contracts, the contractile fibers shorten it to an even larger bulge. For example, extend and then flex your biceps brachii muscle; the large, middle section is the belly. See Figure 7.15[19] for an image of the biceps brachii muscle. When a parallel muscle has a central, large belly that is spindle-shaped, meaning it tapers as it extends to its origin and insertion, it sometimes is called fusiform.
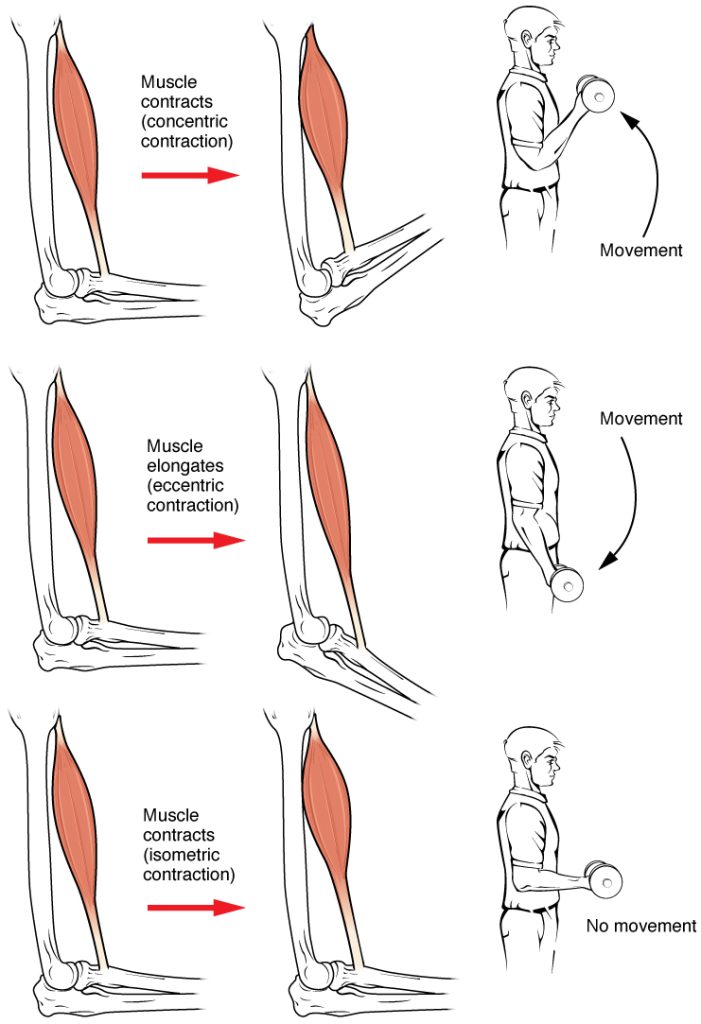
Circular muscles are also called sphincters. When they relax, the sphincters’ circularly arranged bundles of muscle fibers increase the size of the opening, and when they contract, the size of the opening shrinks to close. The orbicularis oris muscle is a circular muscle that goes around the mouth. When it contracts, the oral opening becomes smaller, as when puckering the lips for whistling. Another example is the orbicularis oculi, muscles that surround each eye. Notice the names of the two orbicularis muscles (orbicularis oris and orbicularis oculi), where part of the first name of both muscles is the same. The first part of orbicularis, orb (orb = “circular”), is a reference to a round or circular structure; it may also make one think of orbit, such as the moon’s path around the earth. The word oris (oris = “oral”) refers to the oral cavity, or the mouth. The word oculi (ocular = “eye”) refers to the eye.
There are other muscles throughout the body named by their shape or location. The deltoid is a large, triangular-shaped muscle that covers the shoulder. It is so named because the Greek letter delta looks like a triangle. The rectus abdominis (rector = “straight”) is the straight muscle in the anterior wall of the abdomen, while the rectus femoris is the straight muscle in the anterior compartment of the thigh.
When a muscle has a widespread expansion over a sizable area, but then the fascicles come to a single, common attachment point, the muscle is called convergent. The attachment point for a convergent muscle could be a tendon, an aponeurosis (a flat, broad tendon), or a raphe (a very slender tendon). The large muscle on the chest, the pectoralis major, is an example of a convergent muscle because it converges on the greater tubercle of the humerus via a tendon. The temporalis muscle of the cranium is another. Pennate muscles (penna = “feathers”) blend into a tendon that runs through the central region of the muscle for its whole length, somewhat like the quill of a feather with the muscle arranged similar to the feathers. Due to this design, the muscle fibers in a pennate muscle can only pull at an angle, and as a result, contracting pennate muscles do not move their tendons very far. However, because a pennate muscle generally can hold more muscle fibers within it, it can produce relatively more tension for its size. In a unipennate muscle, the fascicles are located on one side of the tendon. The extensor digitorum of the forearm is an example of a unipennate muscle. A bipennate muscle has fascicles on both sides of the tendon. In some pennate muscles, the muscle fibers wrap around the tendon, sometimes forming individual fascicles in the process. This arrangement is referred to as multipennate. A common example is the deltoid muscle of the shoulder, which covers the shoulder but has a single tendon that inserts on the deltoid tuberosity of the humerus.
See Figure 7.16[20] for an illustration of the seven different muscle shapes.
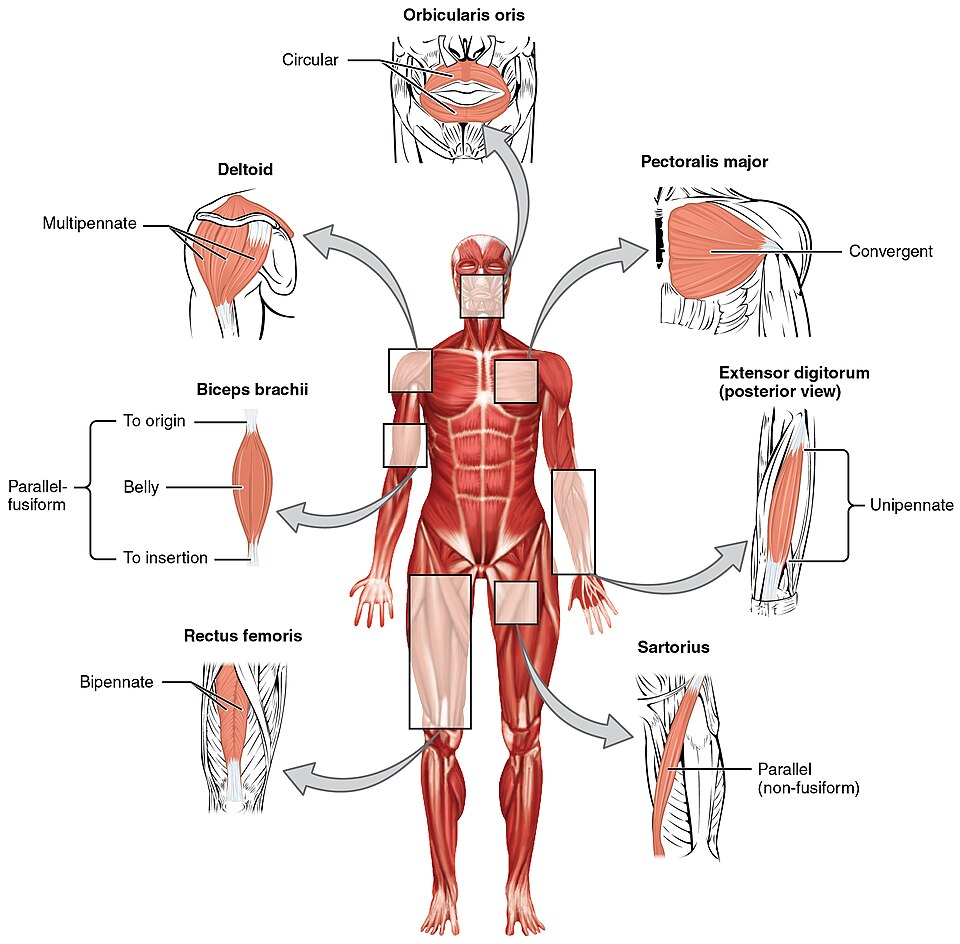
Naming Skeletal Muscles
The Greeks and Romans conducted the first studies done on the human body in Western culture. Subsequent societies studied Latin and Greek, and, therefore, the early pioneers of anatomy continued to apply Latin and Greek terminology or roots when they named the skeletal muscles.
The large number of muscles in the body and unfamiliar words can make learning the names of the muscles in the body seem daunting, but understanding the etymology can help. Etymology is the study of how the root of a particular word entered a language and how the use of the word evolved over time. Taking the time to learn the roots of the words is crucial to understanding the vocabulary of anatomy and physiology. When you understand the names of muscles, it will help you remember where the muscles are located and what they do. See Table 7.3c for some examples of Latin root words and their meanings related to muscles. Table 7.3d provides some mnemonic devices for common Latin roots found in muscle names. Pronunciation of words and terms will take a bit of time to master, but after some basic information, the correct names and pronunciations will become easier.
Table 7.3c. Understanding a Muscle Name from the Latin[21]
Example |
Word |
Latin Root 1 |
Latin Root 2 |
Meaning |
Translation |
---|---|---|---|---|---|
abductor digiti minimi | abductor | ab = away from | duct = to move | A muscle that moves away from | A muscle that moves the little finger or toe away |
digiti | digitus = digit | Refers to a finger or toe | |||
minimi | minimus = mini, tiny | Little | |||
adductor digiti minimi | adductor | ad = to, toward | duct = to move | A muscle that moves toward | A muscle that moves the little finger or toe toward |
digiti | digitus = digit | Refers to a finger or toe | |||
minimi | minimus = mini, tiny | Little |
Table 7.3d. Memory Tips for Latin Roots[22]
Example |
Latin or Greek Translation |
Memory |
---|---|---|
ad | to; toward | ADvance toward your goal. |
ab | away from | To be ABSent from class means being away. |
sub | under | SUBmarines move underwater. |
ductor | something that moves | A conDUCTOR makes a train move. |
anti | against | If you are ANTIsocial, you are against engaging in social activities. |
epi | on top of | The EPIdermis is the top layer of skin |
apo | to the side of | The APOstrophe sits next to the side of a word |
longissimus | longest | “Longissimus” is longer than the word “long.” |
longus | long | A longus muscle is relatively LONG. |
brevis | short | A brevis muscles is relatively short, like a BRIEF meeting. |
maximus | large | “I MAX out” can be used to remember that the gluteus maximus is a large muscle responsible for the extension of the thigh. |
medius | medium | “Medius” and “medium” both begin with “med.” |
minimus | tiny; little | “Mini mouse” can be used to remember that a minimus muscle is tiny or little. |
rectus | straight | To RECTify a situation is to straighten it out. |
multi | many | If something is MULTIcolored, it has many colors. |
uni | one | A UNIcorn has one horn. |
bi/di | two | If a ring is DIcast, it is made of two metals. |
tri | three | TRIple the amount of money is three times as much. |
quad | four | QUADruplets are four children born at one birth. |
externus | outside | An EXTERNal temperature is taken outside of the body. |
internus | inside | An INTERNal temperature is taken inside the body. |
Anatomists name the skeletal muscles according to a number of criteria, each describing the muscle in some way. These include naming the muscle after its shape, its size compared to other muscles in the area, its location in the body or the location of its attachments to the skeleton, how many origins it has, or its action.
The skeletal muscle’s anatomical location or its relationship to a particular bone often determines its name. For example, the frontalis muscle is located anterior to the frontal bone of the skull. Similarly, the shapes of some muscles are very distinctive and the names, such as orbicularis, reflect the shape. For the buttocks, the size of the muscles influences the names: gluteus maximus (largest), gluteus medius (medium), and the gluteus minimus (smallest). Gluteus means buttocks. Names can indicate length—brevis (short) and longus (long)—and identify position relative to the midline—lateralis (away from the midline) and medialis (toward the midline). The direction of the muscle fibers and fascicles are used to describe muscles relative to the midline, such as the rectus (straight) abdominis or the oblique (at an angle) muscles of the abdomen.
Some muscle names indicate the number of muscles in a group. One example of this is the quadriceps femoris, a group of four muscles located on the anterior thigh. Other muscle names can provide information as to how many origins a particular muscle has, such as the biceps brachii. The prefix bi indicates that the muscle has two origins and tri, as in triceps brachii, indicates three origins.
The location of a muscle’s attachment can also appear in its name. When the name of a muscle is based on the attachments, the origin is always named first. For instance, the sternocleidomastoid muscle of the neck has a dual origin on the sternum (sterno) and clavicle (cleido), and it inserts on the mastoid process of the temporal bone.
The last feature by which a muscle is named is its action. When muscles are named for the movement they produce, one can find action words in their name. Some examples are flexor (decreases the angle at the joint), extensor (increases the angle at the joint), abductor (moves the bone away from the midline), or adductor (moves the bone toward the midline).
The skeletal muscles are divided into axial (muscles of the trunk and head) and appendicular (muscles of the arms and legs) categories. This system reflects the bones of the skeletal system, which are also arranged in this manner. The axial muscles are grouped based on location, function, or both.
Major Skeletal Muscles
See Figure 7.17[23] for an illustration of the major skeletal muscles of the human body. Functions of muscles are further described in the following subsections.
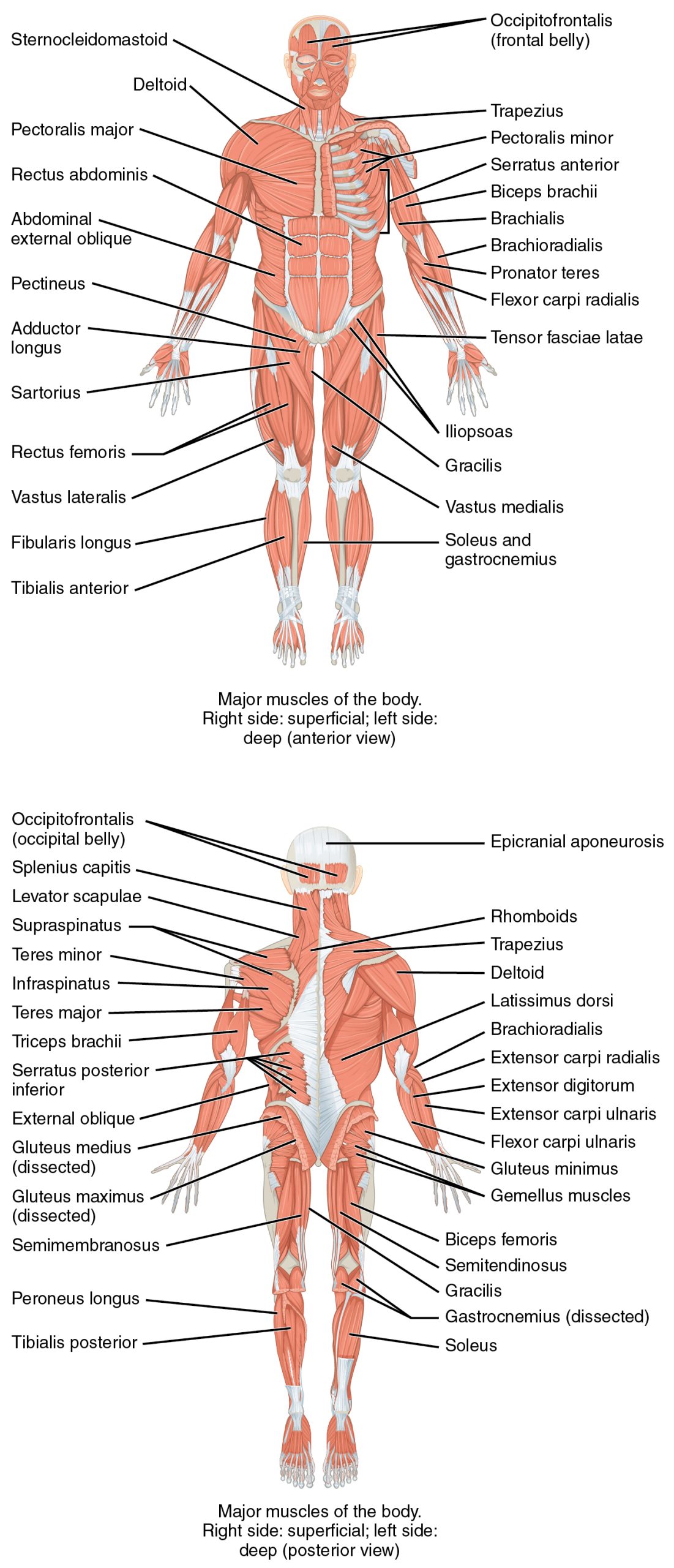
Muscles That Create Facial Expression
The origins of the muscles of facial expression are on the surface of the skull (remember, the origin of a muscle does not move). The insertions of these muscles have fibers intertwined with connective tissue and the dermis of the skin. Because the muscles insert in the skin rather than on bone, when they contract, the skin moves to create facial expression. See Figure 7.18[24] for an illustration of the muscles that create facial expressions.
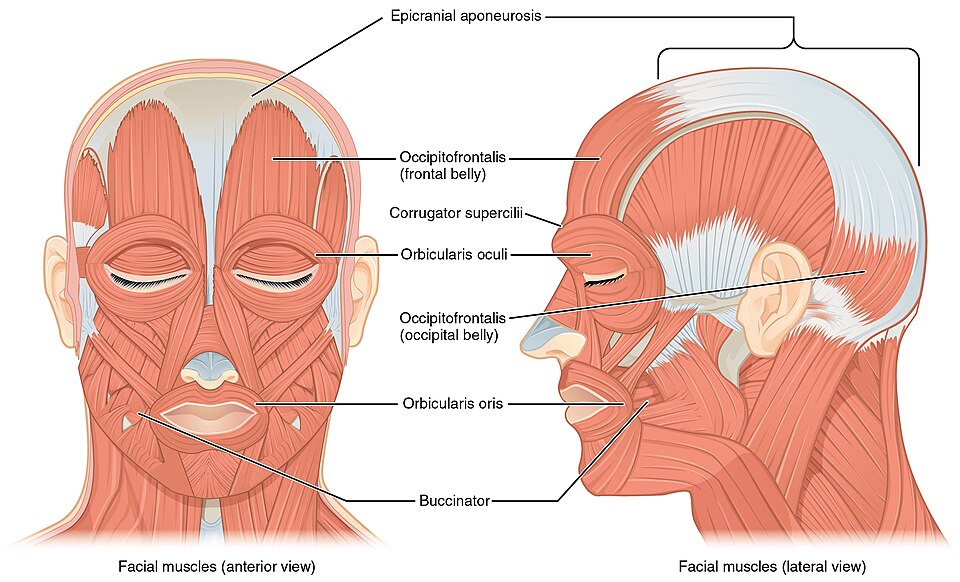
Muscles that create facial expressions include the following:
- Occipitofrontalis: Muscle that raises the eyebrows and wrinkles the forehead. The muscle has a frontal belly and an occipital belly (near the occipital bone on the posterior part of the skull). The muscle on the forehead is the frontalis, and the one on the back of the head is the occipitofrontalis, but there is no muscle across the top of the head. Instead, the two bellies are connected by a broad tendon called the epicranial aponeurosis or galea aponeurosis (galea = “helmet”).
- Orbicularis oris: The circular muscle around the mouth that compresses and puckers the lips.
- Orbicularis oculi: The circular muscle around each eye that closes the eye(s).
- Buccinator: Muscle found in the cheeks that allows you to whistle, blow, and suck. It also contributes to the action of chewing.
- Zygomaticus: Muscle from the zygomatic bone to the mouth. It is the “smiling” muscle.
Muscles That Move the Eyes
- Extrinsic Eye Muscles: The movement of the eyeball is under the control of the extrinsic eye muscles, which originate outside the eye and insert onto the outer surface of the white of the eye. These muscles are located inside the eye socket and cannot be seen on any part of the visible eyeball. See Figure 7.19[25] for an illustration of the extrinsic eye muscles.
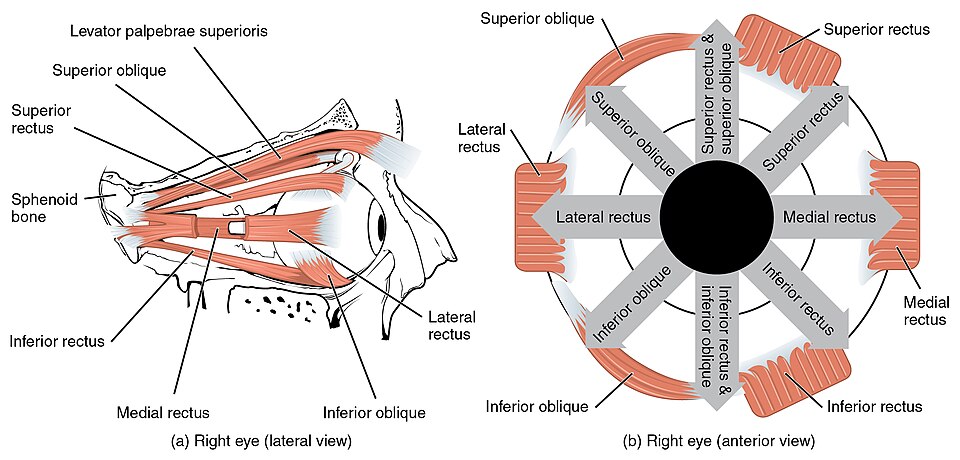
Muscles That Move the Lower Jaw
In anatomical terminology, chewing is called mastication. Muscles involved in chewing must be able to exert enough pressure to bite through and then chew food before it is swallowed. The muscles of mastication include the masseter and the temporalis.
- Masseter: The main muscle used for chewing because it lifts the mandible (lower jaw) to close the mouth.
- Temporalis: The muscle that lifts and pulls back the mandible. You can feel the temporalis move by putting your fingers to your temple as you chew.
See Figure 7.20[26] for an illustration of the masseter and temporalis muscles.

Muscles That Move the Head
The head, attached to the top of the vertebral column, is balanced, moved, and rotated by the neck muscles. When these muscles contract, they can rotate, flex, or extend. The major muscle that moves the head is the sternocleidomastoid.
- Sternocleidomastoid: Major muscle that laterally flexes and rotates the head. When both of them contract, the head flexes at the neck. It has a dual origin on the sternum (stern/o) and clavicle (cleid/o) and inserts on the mastoid process of the temporal bone. Place your fingers on both sides of the neck and turn your head to the left and to the right to feel the movement of this muscle. See Figure 7.21[27] for an illustration of the sternocleidomastoid.
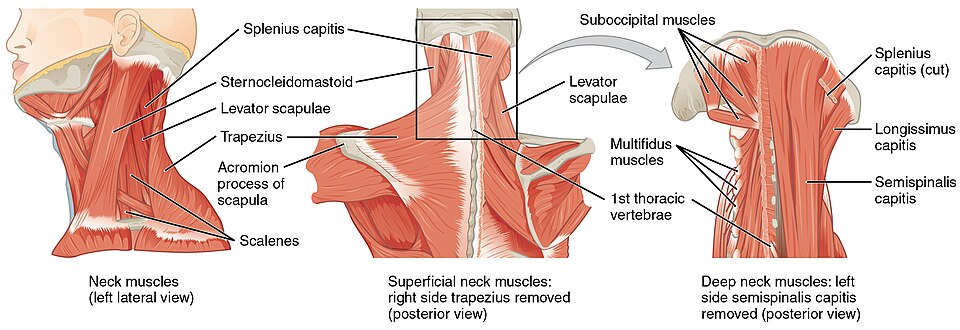
See Table 7.3e in the “Chapter Resources” section for a description of the movements by the muscles that move the head.
Major Muscles of the Abdomen
There are four pairs of abdominal muscles that cover the anterior and lateral abdominal region and meet at the anterior midline. These paired muscles of the anterolateral abdominal wall can be divided into four groups: the external obliques, the internal obliques, the transversus abdominis, and the rectus abdominis. The different orientations of these muscles allow various movements and rotations of the trunk. The three layers of muscle also help to protect the internal abdominal organs in an area where there is no bone.
- External oblique(s): Flat superficial skeletal muscle in the antero-lateral wall of the abdomen. The external oblique is closest to the surface and extends inferiorly and medially, in the direction of sliding one’s four fingers into pants pockets.
- Internal oblique(s): Perpendicular to the external oblique is the intermediate internal oblique, extending superiorly and medially, the direction the thumbs usually go when the other fingers are in the pants pocket.
- Transverse abdominis: The deep muscle is the transversus abdominis. It is arranged transversely around the abdomen, similar to the front of a belt on a pair of pants.
- Rectus abdominis: The straight muscle in the anterior wall of the abdomen, commonly called the “sit-up” muscle. It originates at the pubic crest and symphysis and extends the length of the body’s trunk. The linea alba is a white, fibrous band that is made of the bilateral rectus sheaths that join at the anterior midline of the body. These enclose the rectus abdominis muscles. Each muscle is segmented by three transverse bands of collagen fibers called the tendinous intersections. This results in the look of “six-pack abs,” as each segment hypertrophies on individuals at the gym who do many sit-ups.
- See Figure 7.22[28] for an illustration of the major abdominal muscles.
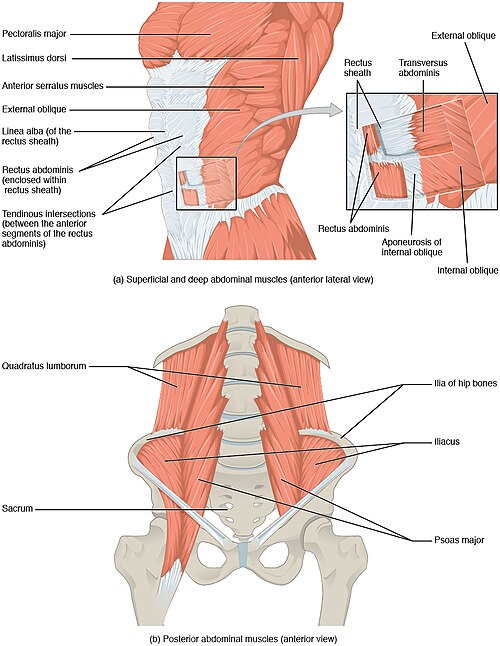
See Table 7.3f in the “Chapter Resources” section for a description of the movements by the abdominal muscles.
Major Muscles of the Chest (Thorax)
The muscles of the chest serve to facilitate breathing by changing the size of the thoracic cavity. When you inhale, your chest rises because the cavity expands. Alternately, when you exhale, your chest falls because the thoracic cavity decreases in size.
Diaphragm
The change in volume of the thoracic cavity during breathing is due to the contraction and relaxation of the diaphragm. See Figure 7.23[29] for an illustration of the diaphragm muscle. The diaphragm separates the thoracic and abdominal cavities and is dome-shaped at rest. The diaphragm also has three openings for structures between the chest cavity and the abdomen. The inferior vena cava passes through the caval opening, and the esophagus and attached nerves pass through the esophageal hiatus. The aorta passes through the aortic hiatus of the posterior diaphragm. See Figure 7.24[30] for an illustration of these openings in the diaphragm.
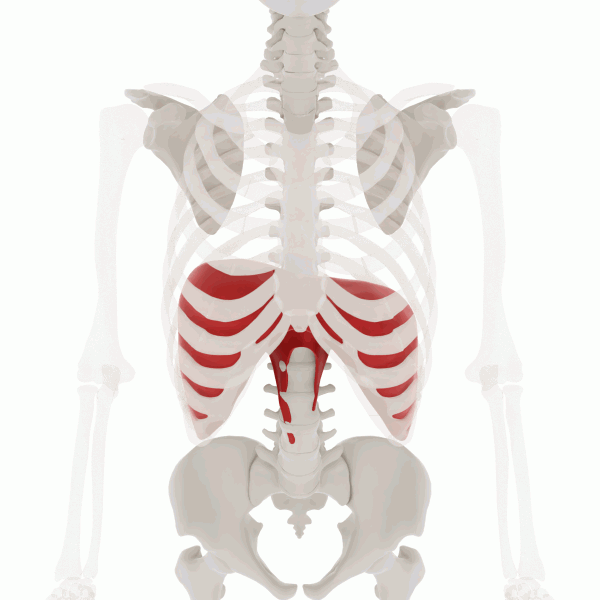
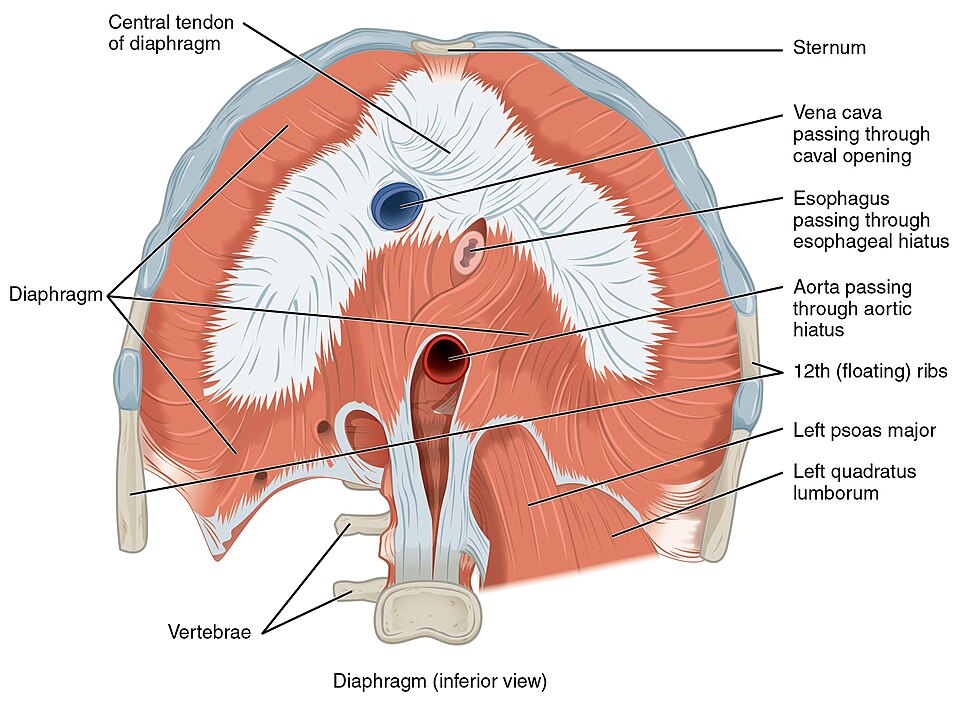
Intercostal Muscles
There are two primary sets of muscles called intercostal muscles, which are found in the intercostal spaces or spaces between the ribs (cost/o = “rib”). The main role of the intercostal muscles is to assist breathing by changing the dimensions of the rib cage. See Figure 7.25[31] for an illustration of the intercostal muscles.
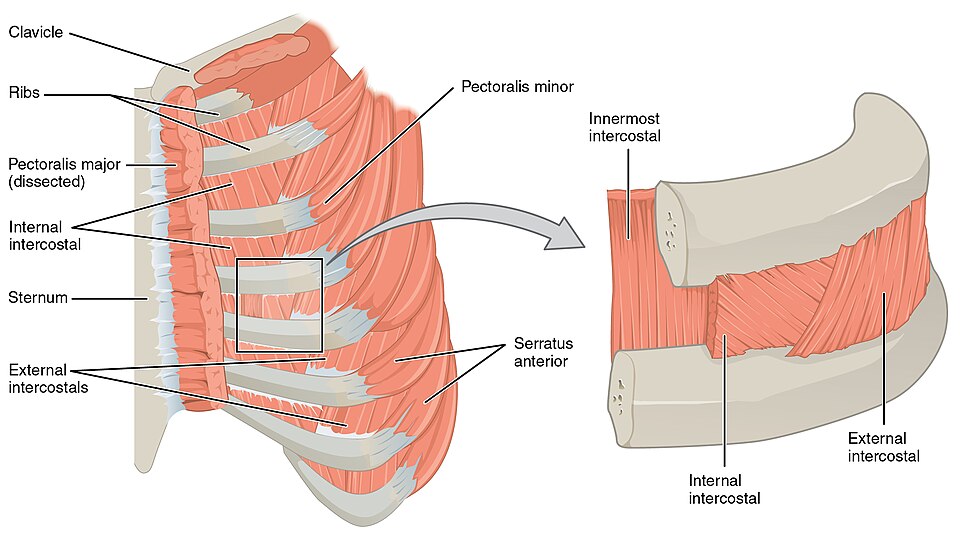
See Table 7.3g in the “Chapter Resources” section for a description of the movements by the thoracic muscles.
Major Muscles of the Shoulder and Upper Limbs
Muscles of the shoulder and upper limb can be divided into four groups: muscles that stabilize and position the pectoral girdle, muscles that move the arm, muscles that move the forearm, and muscles that move the wrists, hands, and fingers.
The pectoral girdle, or shoulder girdle, consists of the lateral ends of the clavicle and scapula, along with the proximal end of the humerus, and the muscles covering these three bones to stabilize the shoulder joint. The girdle creates a base from which the head of the humerus, in its ball-and-socket joint, can move the arm in multiple directions.
Major Muscles of the Pectoral (Shoulder) Girdle
- Pectoralis minor: Rotates shoulder anteriorly (throwing motion)
- Serratus anterior: Moves arm from side of body to front of body
- Trapezius: Elevates shoulders (shrugging), pulls shoulder blades together, and tilts head backwards. The trapezius stabilizes the upper part of the back.
- Rhomboid major: Attaches the vertebral border of the scapula to the spinous process of the thoracic vertebrae. It stabilizes the scapula during pectoral girdle movement.
- Rhomboid minor: Attaches the vertebral border of the scapula to the spinous process of the thoracic vertebrae. It stabilizes the scapula during pectoral girdle movement
See Figure 7.26[32] for an illustration of the pectoral girdle muscles.
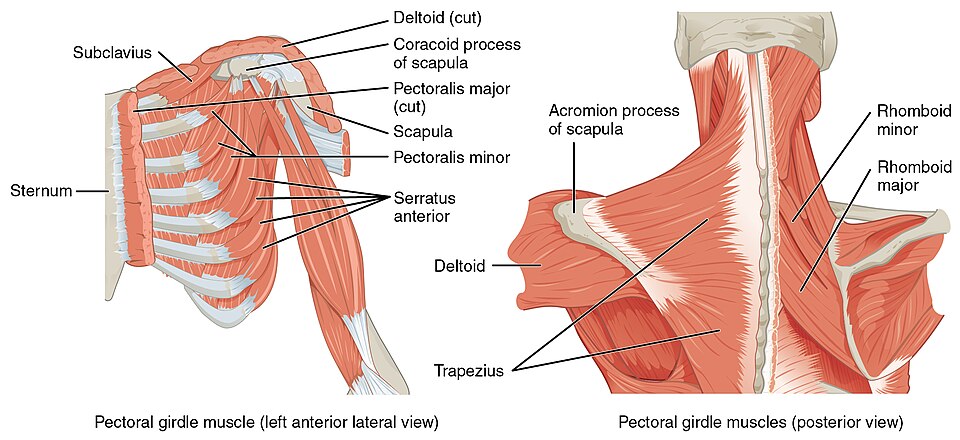
See Table 7.3h in the “Chapter Resources” section for a description of the movements by the muscles that position the pectoral girdle.
Muscles That Move the Humerus
Similar to the muscles that position the pectoral girdle, muscles that cross the shoulder joint and move the humerus bone of the arm include both axial and scapular muscles The two axial muscles are the pectoralis major and the latissimus dorsi. The rest of the shoulder muscles originate on the scapula.
- Pectoralis major: The pectoralis major is thick and fan-shaped, covering much of the superior portion of the anterior thorax. It flexes the arm at the shoulder, adducts, and medially rotates the arm.
- Latissimus dorsi: The broad, triangular latissimus dorsi is located on the inferior part of the back, where it inserts into a thick connective tissue sheath called an aponeurosis. It extends the arm at the shoulder, adducts, and medially rotates the arm.
- Deltoid: The deltoid is the thick muscle that creates the rounded lines of the shoulder and is the major abductor of the arm, but it also facilitates flexing and medial rotation, as well as extension and lateral rotation.
- Subscapularis: The subscapularis originates on the anterior scapula and medially rotates the arm.
- Supraspinatus: The supraspinatus is superior to the spine of the scapula and abducts the arm.
- Infraspinatus: The infraspinatus is inferior to the spine of the scapula and laterally rotates the arm.
- Teres major: The thick and flat teres major is inferior to the teres minor and extends the arm and assists in adduction and medial rotation of it.
- Teres minor: The teres minor laterally rotates, adducts, and extends the arm.
See Figure 7.27[33] for an illustration of muscles that move the humerus.
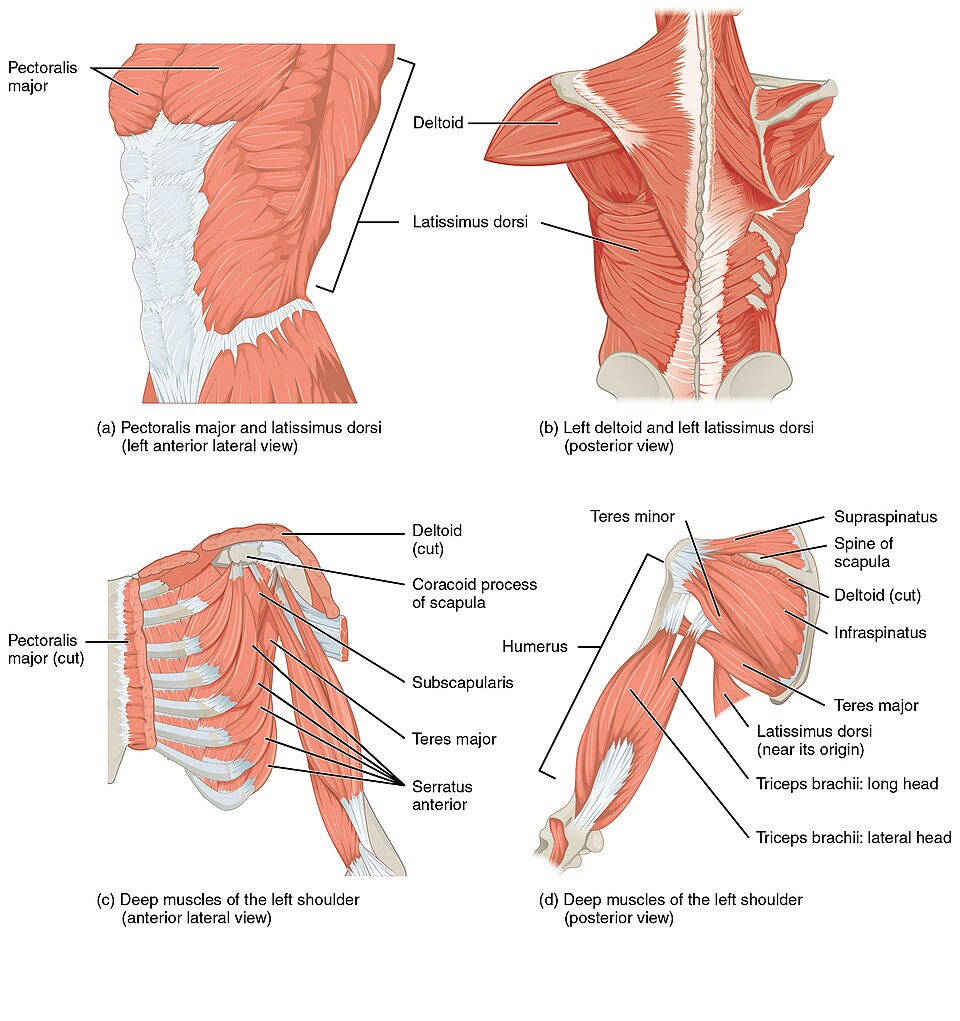
Rotator Cuff
The tendons of the deep subscapularis, supraspinatus, infraspinatus, and teres minor connect the scapula to the humerus, forming the rotator cuff (musculotendinous cuff), the circle of tendons around the shoulder joint. When baseball pitchers undergo shoulder surgery, it is usually on the rotator cuff, which becomes pinched and inflamed and may tear away from the bone due to the repetitive motion of bringing the arm overhead to throw a fast pitch.
See Table 7.3i in the “Chapter Resources” section for a description of the movements by the axial and scapular muscles.
Major Muscles That Move the Forearm
The forearm, made of the radius and ulna bones, has four main types of action at the elbow joint: flexion, extension, pronation, and supination. The major muscles here are as follows:
- Biceps brachii: The two-headed biceps brachii crosses the shoulder and elbow joints to flex the forearm, also taking part in supinating the forearm at the radioulnar joints and flexing the arm at the elbow joint. It performs a biceps curl and also allows the palm of the hand to point toward the body while flexing.
- Brachialis: Deep to the biceps brachii, the brachialis provides additional power in flexing the forearm at the elbow.
- Brachioradialis: The brachioradialis can flex the forearm quickly or help lift a load slowly. When bending the elbow (flexing), the brachioradialis assists the brachialis. The brachioradialis also supinates and pronates the forearm.
- Triceps brachii: The three-headed triceps brachii muscle extends the arm at both the elbow and shoulder joints. Part of it also helps adduct the arm.
See Figure 7.28[34] for an illustration of muscles that move the forearm.
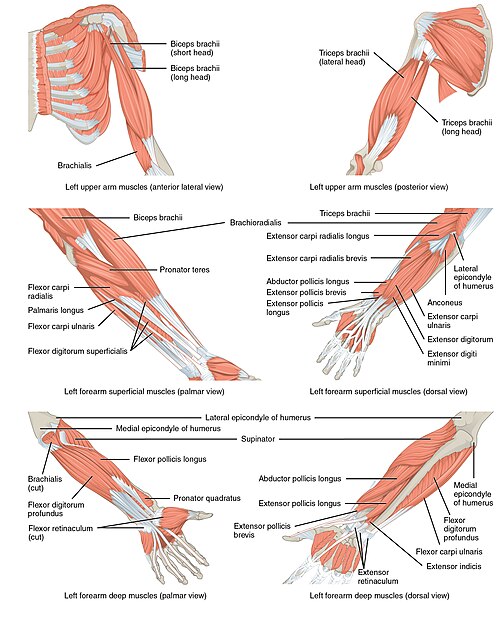
See Table 7.3j in the “Chapter Resources” section for a description of the movements of the muscles that move the forearm.
See Table 7.3k in the “Chapter Resources” section for a description of the movements by the muscles that move the wrist, hands, and forearm.
See Table 7.3l in the “Chapter Resources” section for a description of the movements by the hand muscles.
Muscles of the Pelvis and Lower Limbs
The appendicular muscles of the lower body position and stabilize the pelvic or hip girdle, which serves as a foundation for the lower limbs. The pelvic girdle has less range of motion because it stabilizes and supports the body. The body’s center of gravity is in the area of the pelvis. If the center of gravity were not to remain fixed, standing up would be difficult. Therefore, what the leg muscles lack in range of motion and versatility, they make up for in size and power, facilitating the body’s stabilization, posture, and movement.
Major Muscles of the Upper Leg (Thigh)
Most muscles that insert on and move the femur originate on the pelvic girdle. The muscles found in the hip and thigh include the following:
- Iliopsoas: Muscle group consisting of the iliacus and psoas major muscles, flexing the thigh at the hip, rotating it laterally, and flexing the trunk of the body onto the hip when sitting up from a supine position.
- Gluteus maximus: The largest of the gluteus muscles that extends the femur and laterally rotates the thigh.
- Gluteus medius: The muscle deep to the gluteus maximus that abducts the femur at the hip and medially rotates the thigh.
- Gluteus minimus: Smallest of the gluteal muscles and deep to the gluteus medius. It abducts the hip and medially rotates the thigh.
- Gracilis: The gracilis muscle adducts the thigh in addition to flexing the leg at the knee.
- Quadriceps femoris group: A group of muscles often referred to as the “quads.” It consists of four muscles that extend and stabilize the knee. They move the lower leg out in front of the body, as when kicking. The tendon common to all four is the quadriceps tendon (patellar tendon), which inserts into the proximal part of the patella and continues at the distal area as the patellar ligament. The patellar ligament attaches to the tibial tuberosity.
- Rectus femoris: The rectus femoris is on the anterior aspect of the thigh.
- Vastus lateralis: Found on the lateral aspect of the thigh.
- Vastus medialis: Located on the medial aspect of the thigh.
- Vastus intermedius: Found between vastus lateralis and vastus medialis and deep to the rectus femoris.
- Sartorius: A band-like muscle that extends from the anterior superior iliac spine of the pelvis to the medial side of the proximal tibia. This versatile muscle flexes the leg at the knee and flexes, abducts, and laterally rotates the leg at the hip. This muscle allows us to sit cross-legged.
- Hamstring group: Group of three muscles that flex the knee, extend the hip, and rotate the thigh. The tendons of these muscles form the popliteal fossa, the diamond-shaped space at the back of the knee. You can feel the hamstring tendons behind your knee. The three hamstring muscles include the following:
- Biceps femoris: Muscle located on the lateral side of the posterior thigh.
- Semitendinosus: Muscle located medially next to the biceps femoris.
- Semimembranosus: Most medial muscle of the three from mid-thigh to medial knee. Part of it is deep to semitendinosus.
See Figure 7.29[35] for an illustration of the hip and thigh muscles.
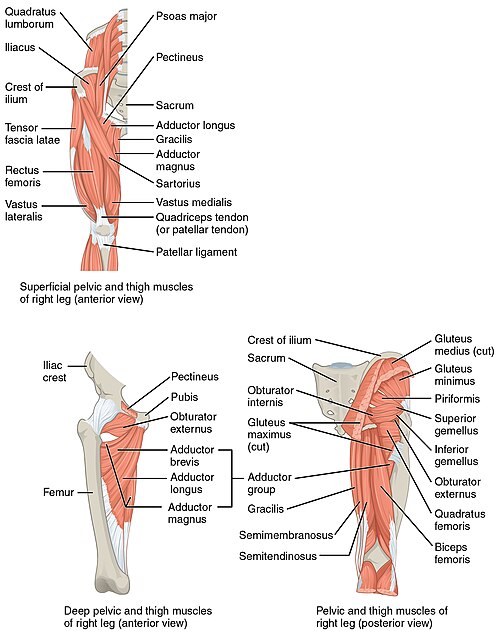
See Table 7.3m in the “Chapter Resources” section for a description of the movements by the gluteal muscles that move the femur.
See Table 7.3n in the “Chapter Resources” section for a description of the movements by the thigh muscles that move the femur, tibia, and fibula.
Major Muscles of the Lower Leg
The lateral and medial muscles of the lower leg invert, evert, and rotate the foot and include the following:
- Tibialis anterior: A long and thick muscle on the anterolateral surface of the tibia. It dorsiflexes and inverts the foot.
- Calcaneal tendon: The calcaneal tendon, also referred to as the Achilles tendon, is a strong tendon that inserts on the calcaneal bone.
- Gastrocnemius: The most superficial and visible muscle of the calf is the gastrocnemius. It plantar flexes the foot and assists in flexing the knee.
- Soleus: Deep to the gastrocnemius is the wide, flat soleus. This muscle also plantar flexes the foot.
See Figure 7.30[36] for an illustration of muscles of the lower leg.
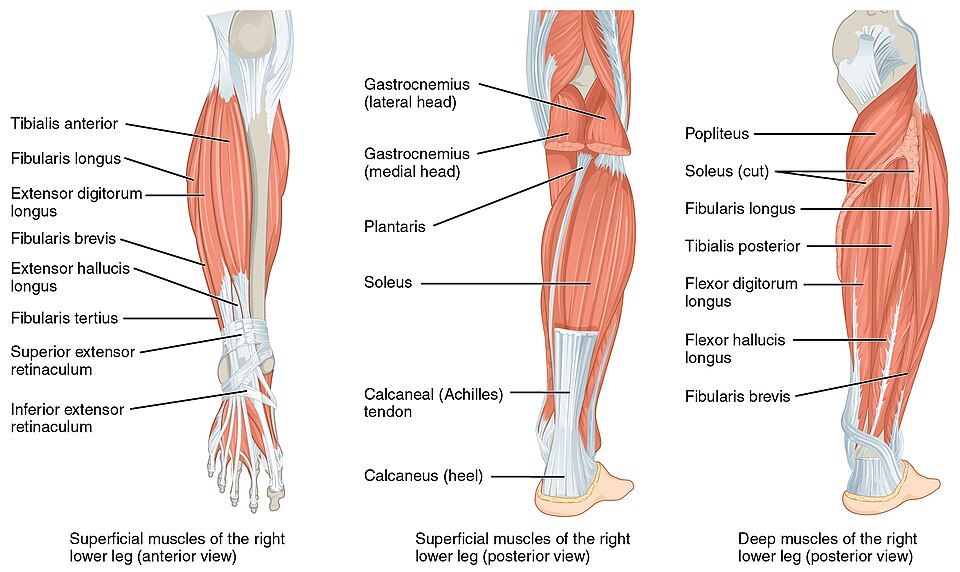
See Figure 7.31[37] for an illustration of the gastrocnemius and soleus.
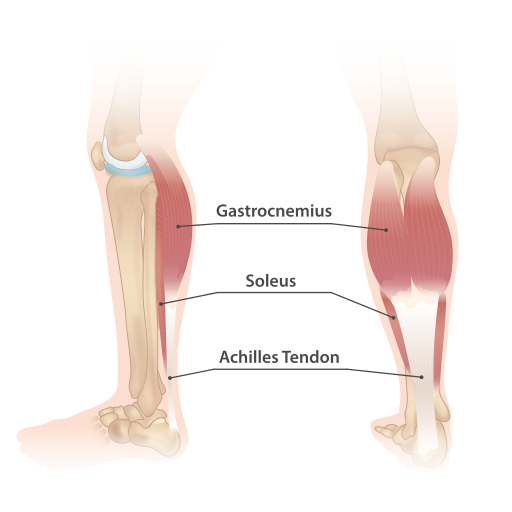
See Table 7.3o in the “Chapter Resources” section for a description of the movements by the muscles that move the feet and toes.
- Betts, J. G., Young, K. A., Wise, J. A., Johnson, E., Poe, B., Kruse, D. H., Korol, O., Johnson, J. E., Womble, M., & DeSaix, P. (2022). Anatomy and Physiology 2e. OpenStax. https://openstax.org/books/anatomy-and-physiology-2e/pages/1-introduction ↵
- “Human Biology fig. 1.20 - Structure of a skeletal muscle - English labels” by National Cancer Institute/SEER Training Modules is in the Public Domain. ↵
- Liang, B. (n.d.). The structure of the muscle organ. Wisc-Online. Licensed under a CC BY NC license. https://www.wisc-online.com/learn/natural-science/life-science/ap13904/the-structure-of-the-muscle-organ ↵
- “1002_Organization_of_Muscle_Fiber” by OpenStax College is licensed under CC BY 4.0 ↵
- “1003_Thick_and_Thin_Filament” by OpenStax College is licensed under CC BY 4.0 ↵
- “Sarcomere” by User:Sameerb is in the Public Domain. ↵
- “Motor_unit” by Daniel Walsh and Alan Sved is licensed under CC BY-SA 4.0 ↵
- “Neuromuscular_junction” by Paul Hege is licensed under CC BY-SA 4.0 ↵
- “运动终板” by Hlj55567516 is licensed under CC BY-SA 3.0 ↵
- “1010a_Contraction_new” by OpenStax College is licensed under CC BY 4.0 ↵
- “1006_Sliding_Filament_Model_of_Muscle_Contraction” by OpenStax College is licensed under CC BY 4.0 ↵
- Amoeba Sisters. (2022, May 5). Muscle tissues and sliding filament model [Video]. YouTube. All rights reserved. https://www.youtube.com/watch?v=dpxalWACO7k ↵
- Allah, B. (2017, May 27). Muscle contraction animation [Video]. YouTube. All rights reserved. https://www.youtube.com/watch?v=Z0qL0v6QFNg ↵
- “1015_Types_of_Contraction_new” by OpenStax is licensed under CC BY 4.0 ↵
- “Biceps_Muscle_CNX” by OpenStax College CC BY-SA 3.0 ↵
- Betts, J. G., Young, K. A., Wise, J. A., Johnson, E., Poe, B., Kruse, D. H., Korol, O., Johnson, J. E., Womble, M., & DeSaix, P. (2022). Anatomy and physiology 2e. OpenStax. https://openstax.org/books/anatomy-and-physiology-2e/pages/1-introduction ↵
- “Body_Movements_I.jpg” by Tonye Ogele CNX is licensed under CC BY-SA 3.0 ↵
- “Body_Movements_II.jpg” by Tonye Ogele CNX is licensed under CC BY-SA 3.0 ↵
- “Biceps_Brachii_Muscle_Contraction” by OpenStax College is licensed under CC BY-SA 3.0 ↵
- “Fascicle_Muscle_Shapes” by OpenStax College is licensed under CC BY-SA 3.0 ↵
- Betts, J. G., Young, K. A., Wise, J. A., Johnson, E., Poe, B., Kruse, D. H., Korol, O., Johnson, J. E., Womble, M., & DeSaix, P. (2022). Anatomy and physiology 2e. OpenStax. https://openstax.org/books/anatomy-and-physiology-2e/pages/1-introduction ↵
- Betts, J. G., Young, K. A., Wise, J. A., Johnson, E., Poe, B., Kruse, D. H., Korol, O., Johnson, J. E., Womble, M., & DeSaix, P. (2022). Anatomy and physiology 2e. OpenStax. https://openstax.org/books/anatomy-and-physiology-2e/pages/1-introduction ↵
- “1105_Anterior_and_Posterior_Views_of_Muscles” by OpenStax is licensed under CC BY 4.0 ↵
- “1106_Front_and_Side_Views_of_the_Muscles_of_Facial_Expressions” by OpenStax is licensed under CC BY 4.0 ↵
- “1107_The_Extrinsic_Eye_Muscles” by OpenStax is licensed under CC BY 4.0 ↵
- “1108_Muscle_that_Move_the_Lower_Jaw” by OpenStax is licensed under CC BY 4.0 ↵
- “1111_Posterior_and_Side_Views_of_the_Neck” by OpenStax is licensed under CC BY 4.0 ↵
- “1112_Muscles_of_the_Abdomen” by OpenStax is licensed under CC BY 4.0 ↵
- “Human_diaphragm.png” by Anatomography is licensed under CC BY-SA 2.1 Japan ↵
- “1113_The_Diaphragm” by OpenStax is licensed under CC BY 4.0 ↵
- “1114_Thorax” by OpenStax is licensed under CC BY 4.0 ↵
- “1118_Muscles_that_Position_the_Pectoral_Girdle” by OpenStax is licensed under CC BY 4.0 ↵
- “1119_Muscles_that_Move_the_Humerus” by OpenStax is licensed under CC BY 4.0 ↵
- “1120_Muscles_that_Move_the_Forearm” by OpenStax is licensed under CC BY 4.0 ↵
- “1122_Gluteal_Muscles_that_Move_the_Femur” by OpenStax is licensed under CC BY 4.0 ↵
- “1123_Muscles_of_the_Leg_that_Move_the_Foot_and_Toes” by OpenStax is licensed under CC BY 4.0 ↵
- “Lower_leg_muscles” by InjuryMap is licensed under CC BY-SA 4.0 ↵
Striated muscle tissue attached to bones that enables movement, facial expressions, posture, and other voluntary actions.
Groups of axons bundled within a nerve.
A connective tissue layer that surrounds and protects skeletal muscles.
Connective tissue that surrounds individual muscle fascicles within a muscle.
Thin connective tissue that surrounds individual muscle fibers within a fascicle.
A fibrous connective tissue that attaches muscle to bone.
A flat, broad connective tissue that connects muscles to each other or to bones.
A fibrous tissue that encloses muscles and organs.
Elongated cells that make up muscle tissue, responsible for contraction and movement.
Cell membrane that surrounds a muscle fiber, playing a crucial role in conducting electrical impulses and maintaining the fiber's structural integrity.
The cytoplasm of a muscle fiber, containing various organelles, myofibrils, and energy stores, such as glycogen and myoglobin, essential for muscle function.
A specialized form of the endoplasmic reticulum in muscle cells that stores and regulates calcium ions, crucial for muscle contraction and relaxation.
Long, thread-like structures within muscle fibers that contain the contractile proteins actin and myosin, responsible for muscle contraction. Myofibrils are arranged in a repeating pattern, forming sarcomeres, the functional units of muscle.
The basic functional unit of a muscle fiber, defined as the segment between two Z discs. Sarcomeres contain overlapping thin (actin) and thick (myosin) filaments, which interact to produce muscle contraction.
A protein that forms thin filaments in muscle fibers, essential for muscle contraction through its interaction with myosin.
A motor protein that forms thick filaments in muscle fibers, responsible for muscle contraction by interacting with actin to generate force.
Composed primarily of actin, these filaments are thinner than myosin and are referred to as thin filaments in the sarcomere. They play a key role in muscle contraction by interacting with thick filaments (myosin).
Composed primarily of myosin, these filaments are thicker than actin and are responsible for generating force during muscle contraction by interacting with thin filaments in the sarcomere.
Responsible for muscle contraction, with the number of fibers influenced by the precision of movement required.
Process by which an increase in the number of active motor units enhances muscle contraction strength.
The synapse or connection point between a motor neuron and a muscle fiber, where the transmission of signals occurs to initiate muscle contraction.
Charged particles (positively or negatively) that are essential for cellular processes, such as nerve signaling and muscle contraction (e.g., Na⁺, K⁺, Ca²⁺, Cl⁻).
Links electrical signals to muscle contraction.
Rapid electrical signals.
Chemical messengers.
Neurotransmitter that plays a key role in muscle contraction and communication between neurons in the nervous system.
Small gap between a motor neuron and the muscle fiber's sarcolemma.
Specialized region of the sarcolemma at the neuromuscular junction where ACh receptors are concentrated.
The process by which the membrane potential of a cell becomes less negative.
Membrane proteins that open in response to changes in electrical potential.
The process by which a cell's membrane potential returns to its resting state after depolarization, typically involving the efflux of potassium ions from the cell.
Actin and myosin filaments slide past each other to shorten the sarcomere, resulting in muscle fiber shortening and contraction.
Increased mass and bulk in a skeletal muscle.
Decrease in the size and strength (but not the number) of muscle fibers.
Force generated by the contraction of the muscle (or shortening of the sarcomeres).
Muscle contractions that involve a change in muscle length while maintaining a constant tension.
Type of muscle contraction where the muscle shortens while generating tension, typically occurring during the lifting phase of a movement.
Type of muscle contraction where the muscle lengthens while generating tension, typically occurring during the lowering phase of a movement.
Type of muscle contraction where the muscle generates tension without changing length, maintaining a static position.
Constant, low-level contraction of muscles that maintains posture and stability, regulated by the nervous system.
Absence of the low-level contractions that lead to muscle tone.
Excessive muscle tone.
An increase in muscle tone and stiffness.
The moveable end of a muscle that attaches to the bone being pulled.
The end of the muscle attached to a fixed (stabilized) bone.
A muscle that plays the primary role in producing a specific movement. Also known as an "agonist.
A muscle that contracts to produce a specific movement. Also known as the "prime mover.
A muscle that assists the agonist (prime mover) in performing a specific movement.
Stabilizes a joint or body part, allowing the agonist to perform its action more effectively.
Muscle with the opposite action of the prime mover.
Type of muscle architecture where the muscle fibers run parallel to the long axis of the muscle.
The central, fleshy part of a muscle, which typically contains the bulk of muscle fibers.
A type of muscle shape characterized by a tapered end and a wider belly, resembling a spindle.
Muscles that are arranged in a circular pattern around an opening or a surface.
Circular muscles that encircle and control the opening of various passages in the body.
Muscles that have a broad origin and converge to a single tendon or point of insertion.
Very slender tendon.
Muscles characterized by a feather-like arrangement of fibers
Fascicles are located on one side of the tendon.
Fascicles on both sides of the tendon.
Multiple tendons with muscle fibers branching out from each side.
Muscles that are large or the largest in a group.
Muscles that are moderate or medium-sized.
Muscles that are small in size.
Muscles that are short in length.
Muscles that are elongated or long in shape.
Muscles located away from the midline of the body.
Muscles located toward the midline of the body.
Muscles characterized by their straight alignment.
Fracture that occurs at an angle that is not 90 degrees.
Muscle that raises the eyebrows and wrinkles the forehead
Muscle on the forehead.
Muscle on the back of the head.
Circular muscle around the mouth that compresses and puckers the lips
Circular muscle around each eye that closes the eye(s)
Muscle found in the cheeks that allows you to whistle, blow, and suck.
Muscle from the zygomatic bone to the mouth. The “smiling’ muscle.
Controls the movement of the eyeball.
Anatomical term for chewing.
Main muscle used for chewing.
Muscle that lifts and pulls back the mandible.
Major muscle that laterally flexes and rotates the head.
Flat superficial skeletal muscle in the antero-lateral wall of the abdomen
Perpendicular to the external oblique is the intermediate internal oblique, extending superiorly and medially, the direction the thumbs usually go when the other fingers are in the pants pocket
The deep muscle is the transversus abdominis
The straight muscle in the anterior wall of the abdomen, commonly called the “sit-up” muscle
Fibrous strip in the middle of the abdomen where the abdominal muscles meet.
Fibrous coverings that enclose the rectus abdominis muscles.
Fibrous bands that divide the rectus abdominis muscle into segments, creating the "six-pack" appearance.
Separates the thoracic and abdominal cavities and is dome-shaped at rest.
Opening in the diaphragm that allows the inferior vena cava (a large vein) to pass from the abdomen into the chest cavity.
Opening in the diaphragm through which the esophagus passes from the thoracic cavity into the abdominal cavity.
Opening in the diaphragm that allows the aorta, the body's main artery, to pass from the thoracic cavity into the abdominal cavity.
A group of muscles located between the ribs.
Area which serves to attach the upper limb to the sternum of the axial skeleton, consisting of the clavicle and the scapula.
Rotates shoulder anteriorly (throwing motion).
Moves arm from side of body to front of body.
Elevates shoulders (shrugging); pulls shoulder blades together; tilts head backwards.
Attaches the vertebral border of the scapula to the spinous process of the thoracic vertebrae.
Attaches the vertebral border of the scapula to the spinous process of the thoracic vertebrae.
A large, fan-shaped muscle in the chest that aids in shoulder movement.
The broad, triangular latissimus dorsi is located on the inferior part of the back, where it inserts into a thick connective tissue sheath called an aponeurosis.
Thick muscle that creates the rounded lines of the shoulder and is the major abductor of the arm, but it also facilitates flexing and medial rotation, as well as extension and lateral rotation.
A large muscle located on the anterior surface of the scapula, responsible for internal rotation and adduction of the shoulder.
A shoulder muscle located above the scapula that initiates arm abduction and stabilizes the shoulder joint.
Inferior to the spine of the scapula and laterally rotates the arm.
Thick and flat; inferior to the teres minor and extends the arm and assists in adduction and medial rotation.
Laterally rotates, adducts, and extends the arm.
Circle of tendons around the shoulder joint.
A muscle located in the upper arm. It has two main parts (or "heads") and is responsible for bending the elbow and rotating the forearm.
Deep to the biceps brachii, the brachialis provides additional power in flexing the forearm at the elbow.
A muscle of the forearm that helps flex the elbow.
A muscle located at the back of the upper arm. It has three heads (points of origin) and is responsible for straightening the elbow.
Serves as a foundation for the lower limbs.
Muscle group consisting of the iliacus and psoas major muscles, flexes the thigh at the hip, rotates it laterally, and flexes the trunk of the body onto the hip when sitting up from a supine position.
The largest of the gluteus muscles that extends the femur and laterally rotates the thigh.
The muscle deep to the gluteus maximus that abducts the femur at the hip and medially rotates the thigh.
Smallest of the gluteal muscles and deep to the gluteus medius.
Adducts the thigh in addition to flexing the leg at the knee.
A group of muscles often referred to as the “quads,” it consists of four muscles that extend and stabilize the knee.
Connects the quadriceps muscle group (located in the front of the thigh) to the patella (kneecap).
Connects the patella (kneecap) to the tibia (shinbone).
On the anterior aspect of the thigh.
On the lateral aspect of the thigh.
On the medial aspect of the thigh.
Between vastus lateralis and vastus medialis and deep to the rectus femoris.
A band-like muscle that extends from the anterior superior iliac spine of the pelvis to the medial side of the proximal tibia.
Group of three muscles that flex the knee, extend the hip and rotate the thigh.
Diamond-shaped space at the back of the knee.
Muscle located on the lateral side of the posterior thigh.
Muscle located medially next to the biceps femoris.
Most medial muscle of the three from mid-thigh to medial knee. Part of it is deep to semitendinosus.
Long and thick muscle on the anterolateral surface of the tibia.
Also referred to as the Achilles tendon, is a strong tendon that inserts on the calcaneal bone.
The most superficial and visible muscle of the calf.
Deep to the gastrocnemius is the wide, flat soleus.